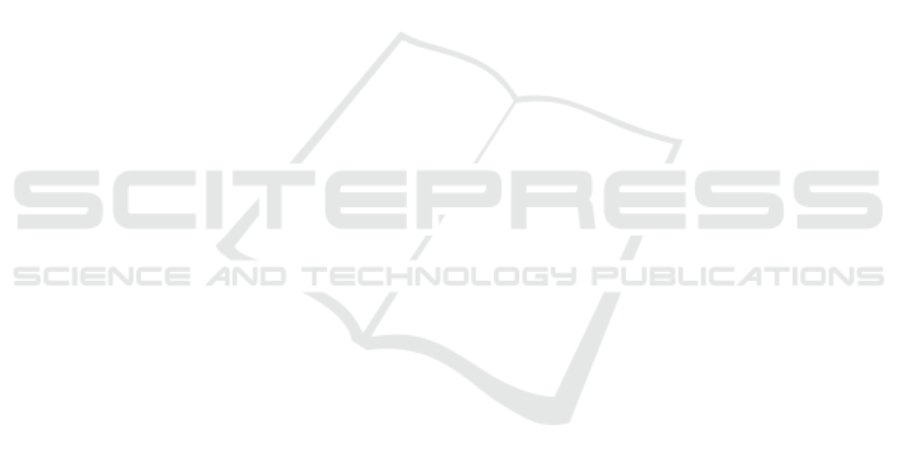
rather than reactive responses to potential grid chal-
lenges.
Communication networks facilitate the integra-
tion of decentralized energy resources (DERs), mi-
crogrids, and renewable energy sources, which are
increasingly essential in the transition to a more sus-
tainable and resilient energy system. Reliable connec-
tivity ensures that these distributed assets can operate
harmoniously within the larger grid.
Strengthening grid communication infrastructure
must be prioritized in energy policies and investment
strategies. Governments, utilities, and stakeholders
need to collaborate on standardizing communication
technologies and ensuring interoperability to enhance
resilience on a global scale.
Enabling intelligent decision-making at the edges
of the power grid, instead of relying solely on con-
trol centers, is important, especially during extreme
weather events were the connection to the control cen-
ter can fail.
Addressing grid resilience in the context of cli-
mate change requires a holistic approach, combining
robust communication networks with smart grid tech-
nologies, predictive analytics, and sustainable energy
integration. This approach will ensure power grids
remain reliable, efficient, and adaptive in the face of
evolving climate challenges.
Communication networks are no longer a sec-
ondary consideration but a cornerstone of modern,
climate-resilient power grids. Investing in advanced,
secure, and resilient communication technologies is
essential to meeting the dual challenges of climate
adaptation and energy transition.
ACKNOWLEDGEMENTS
The presented work is conducted in the research
project INFRADAPT which is funded by the Austrian
Climate and Energy Fund and is being carried out as
part of the Energy Research Programme 2022.
REFERENCES
Abrahamsen, F. E., Ai, Y., and Cheffena, M. (2021). Com-
munication Technologies for Smart Grid: A Compre-
hensive Survey. Sensors, 21(23):8087.
Bhat, S. (2024). Leveraging 5G Network Capabilities for
Smart Grid Communication. Journal of Electrical
Systems, 20(2):2272–2283.
Caldeira, R. T., Melgarejo, D. C., and Coutinho, R. (2017).
Application of LTE 450 MHz in the electric energy
sector. In 2017 European Conference on Networks
and Communications (EuCNC), pages 1–5, Oulu, Fin-
land. IEEE.
Carvallo, J. P. C. and Casey, J. (2024). Surging Power Out-
ages and Climate Change. Technical report, Climate
Central.
Crivellaro, A., Tayyebi, A., Gavriluta, C., Gross, D., Anta,
A., Kupzog, F., and Dorfler, F. (2020). Beyond low-
inertia systems: Massive integration of grid-forming
power converters in transmission grids. In 2020 IEEE
Power & Energy Society General Meeting (PESGM),
pages 1–5, Montreal, QC, Canada. IEEE.
European 5G Observatory (2024). 5G Private net-
works – 5G Observatory. https://5gobservatory.eu/
5g-private-networks/.
European Commission. Joint Research Centre. (2020). Cli-
mate change impacts and adaptation in Europe: JRC
PESETA IV final report. Publications Office, LU.
Gamboa, S., Ben Mosbah, A., Garey, W., Liu, C., and Rouil,
R. (2023). System-Level Evaluation of 5G NR UE-
Based Relays. In MILCOM 2023 - 2023 IEEE Mil-
itary Communications Conference (MILCOM), pages
807–814, Boston, MA, USA. IEEE.
Gonc¸alves, A. C. R., Costoya, X., Nieto, R., and Liberato,
M. L. R. (2024). Extreme weather events on energy
systems: a comprehensive review on impacts, miti-
gation, and adaptation measures. Sustainable Energy
Research, 11(1):4.
Horrocks, L., Beckford, J., Hodgson, N., Downing, C.,
Davey, R., and O’Sullivan, A. (2010). Adapting the
ICT Sector to the Impacts of Climate Change – Final
Report. Technical report, AEA group.
Hou, X., Wild, M., Folini, D., Kazadzis, S., and Wohland, J.
(2021). Climate change impacts on solar power gen-
eration and its spatial variability in europe based on
cmip6. Earth System Dynamics, 12(4):1099–1113.
Kamwa, I. and Johnson, B. K. (2023). Grid communica-
tions: The wide-area control and situational awareness
[Editors’ Voice]. IEEE Power and Energy Magazine,
21(5):4–13.
Khalid, S., Hotovec, S., and Clancy, J. (2023). Utilities’
Need for Advanced Telecommunications. Climate
and Energy, 39(6):1–10.
Pedro, H. T. and Coimbra, C. F. (2012). Assessment of fore-
casting techniques for solar power production with no
exogenous inputs. Solar Energy, 86(7):2017–2028.
Purushottam Kumar Maurya (2024). Self-Healing Grids:
AI Techniques for Automatic Restoration after Out-
ages. Power System Technology, 48(1):494–510.
Ward, D. M. (2013). The effect of weather on grid sys-
tems and the reliability of electricity supply. Climatic
Change, 121(1):103–113.
The Increasingly Critical Role of Communication Networks in Enhancing Power Grid Resilience Under Climate Change
187