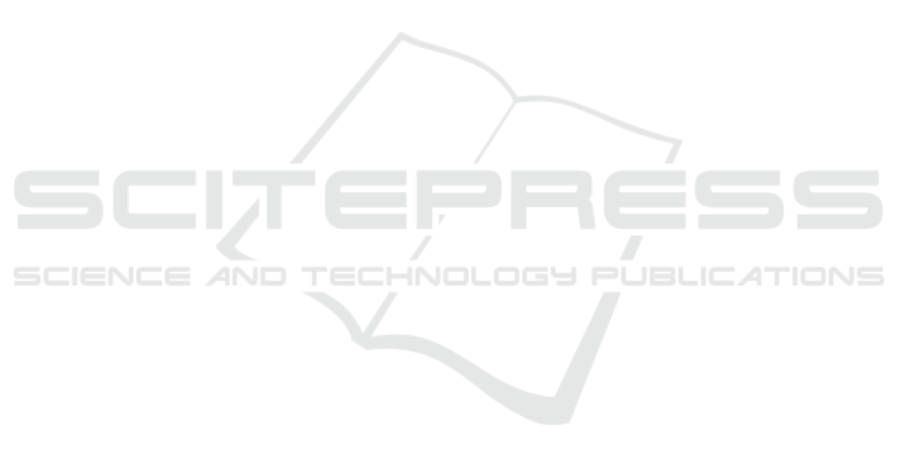
6.1 Limitations and Future Work
Our implementation of the knob manipulation is sen-
sitive to hand motions. That caused some overshoots
and additional errors, when participants were placing
the cube to the target position. This can be improved
in two possible ways: either by decreasing the sensi-
tivity of the knob by increasing the angular resolution,
or by creating a slightly bigger target area to avoid
those overshoots that are caused by a small hand mo-
tion before the release of the virtual knob.
REFERENCES
Apostolou, K. and Liarokapis, F. (2022). A systematic re-
view: The role of multisensory feedback in virtual
reality. In 2022 IEEE 2nd International Conference
on Intelligent Reality (ICIR), pages 39–42, New York,
NY, USA. IEEE.
Bermejo, C., Lee, L. H., Chojecki, P., Przewozny, D., and
Hui, P. (2021). Exploring button designs for mid-
air interaction in virtual reality: A hexa-metric eval-
uation of key representations and multi-modal cues.
Proceedings of the ACM on Human-Computer Inter-
action, 5(EICS):1–26.
Brooke, J. (1996). Sus: a “quick and dirty’usability. Us-
ability evaluation in industry, 189(3):189–194.
Cooper, N., Milella, F., Pinto, C., Cant, I., White, M., and
Meyer, G. (2018). The effects of substitute multisen-
sory feedback on task performance and the sense of
presence in a virtual reality environment. PloS one,
13(2):e0191846.
Faeth, A. and Harding, C. (2014). Emergent effects in mul-
timodal feedback from virtual buttons. ACM Trans-
actions on Computer-Human Interaction (TOCHI),
21(1):1–23.
Gallace, A., Ngo, M. K., Sulaitis, J., and Spence, C. (2012).
Multisensory presence in virtual reality: possibilities
& limitations. In Multiple sensorial media advances
and applications: New developments in MulSeMedia,
pages 1–38. IGI Global, Hershey, PA, USA.
Hart, S. G. and Staveland, L. E. (1988). Development of
nasa-tlx (task load index): Results of empirical and
theoretical research. In Advances in psychology, vol-
ume 52, pages 139–183. Elsevier, Paris, France.
Hinricher, N., Schr
¨
oer, C., and Backhaus, C. (2023). Design
of control elements in virtual reality—investigation
of factors influencing operating efficiency, user ex-
perience, presence, and workload. Applied Sciences,
13(15):8668.
Hirt, C., Spahni, M., Kompis, Y., Jetter, D., and Kunz, A.
(2020). Virtual reality training platform for a com-
puter numerically controlled grinding machine tool.
International Journal of Mechatronics and Manufac-
turing Systems, 14(1):1–17.
Kennedy, R. S., Lane, N. E., Berbaum, K. S., and Lilien-
thal, M. G. (1993). Simulator sickness questionnaire:
An enhanced method for quantifying simulator sick-
ness. The international journal of aviation psychol-
ogy, 3(3):203–220.
Kreimeier, J., Hammer, S., Friedmann, D., Karg, P., B
¨
uhner,
C., Bankel, L., and G
¨
otzelmann, T. (2019). Evaluation
of different types of haptic feedback influencing the
task-based presence and performance in virtual real-
ity. In Proceedings of the 12th acm international con-
ference on pervasive technologies related to assistive
environments, pages 289–298, New York, NY, USA.
ACM.
Lefor, A. K., Harada, K., Kawahira, H., and Mitsuishi, M.
(2020). The effect of simulator fidelity on procedure
skill training: a literature review. International jour-
nal of medical education, 11:97.
Man, D. and Olchawa, R. (2018). The possibilities of using
bci technology in biomedical engineering. In Biomed-
ical Engineering and Neuroscience: Proceedings of
the 3rd International Scientific Conference on Brain-
Computer Interfaces, BCI 2018, March 13-14, Opole,
Poland, pages 30–37, Cham, Switzerland. Springer.
Marucci, M., Di Flumeri, G., Borghini, G., Sciaraffa, N.,
Scandola, M., Pavone, E. F., Babiloni, F., Betti, V., and
Aric
`
o, P. (2021). The impact of multisensory integra-
tion and perceptual load in virtual reality settings on
performance, workload and presence. Scientific Re-
ports, 11(1):4831.
Matthews, B. J., Thomas, B. H., Von Itzstein, G. S., and
Smith, R. T. (2023). Towards applied remapped
physical-virtual interfaces: Synchronization methods
for resolving control state conflicts. In Proceedings of
the 2023 CHI Conference on Human Factors in Com-
puting Systems, pages 1–18, New York, NY, USA.
ACM.
Slater, M. et al. (1999). Measuring presence: A re-
sponse to the witmer and singer presence question-
naire. Presence: teleoperators and virtual environ-
ments, 8(5):560–565.
Tatzgern, M. and Birgmann, C. (2021). Exploring input
approximations for control panels in virtual reality.
In 2021 IEEE Virtual Reality and 3D User Interfaces
(VR), pages 1–9, New York, NY, USA. IEEE.
Multimodal Feedback Estimation for Knob Interactions in Virtual Reality for Control Panels
595