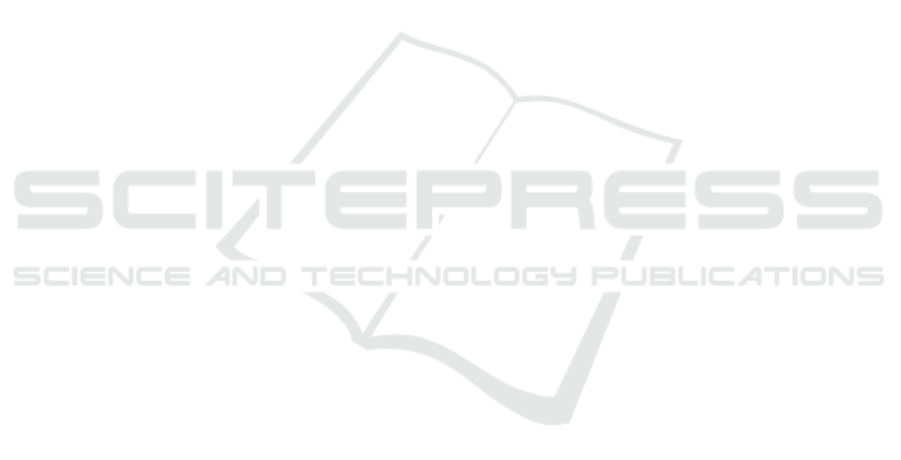
augmented reality-guided surgery: Preclinical phan-
tom evaluation for high-precision maxillofacial tasks.
Journal of Clinical Medicine, 9:3562.
Cercenelli, L., Emiliani, N., Gulotta, C., Bevini, M., Badi-
ali, G., and Marcelli, E. (2023). Augmented reality in
orthognathic surgery: A multi-modality tracking ap-
proach to assess the temporomandibular joint motion.
Condino, S., Cutolo, F., Carbone, M., Cercenelli, L., Badi-
ali, G., Montemurro, N., and Ferrari, V. (2023). Reg-
istration sanity check for ar-guided surgical interven-
tions: Experience from head and face surgery. IEEE
Journal of Translational Engineering in Health and
Medicine, pages 1–1.
Fida, B., Cutolo, F., di Franco, G., Ferrari, M., and Ferrari,
V. (2018). Augmented reality in open surgery. Up-
dates in Surgery, 70:389–400.
Fitzpatrick, J. M. (2010). The role of registration in accurate
surgical guidance. Proceedings of the Institution of
Mechanical Engineers, Part H: Journal of Engineer-
ing in Medicine, 224:607–622.
Fotouhi, J., Alexander, C. P., Unberath, M., Taylor, G.,
Lee, S. C., Fuerst, B., Johnson, A., Osgood, G., Tay-
lor, R. H., Khanuja, H., Armand, M., and Navab,
N. (2018). Plan in 2-d, execute in 3-d: an aug-
mented reality solution for cup placement in total hip
arthroplasty. Journal of medical imaging (Bellingham,
Wash.), 5:021205.
Fraga-Lamas, P., Fernandez-Carames, T. M., Blanco-
Novoa, O., and Vilar-Montesinos, M. A. (2018). A
review on industrial augmented reality systems for the
industry 4.0 shipyard. IEEE Access, 6:13358–13375.
Friedman, M. (1937). The use of ranks to avoid the as-
sumption of normality implicit in the analysis of vari-
ance. Journal of the American Statistical Association,
32:675–701.
Friedman, M. (1939). A correction. Journal of the Ameri-
can Statistical Association, 34:109–109.
Friedman, M. (1940). A comparison of alternative tests of
significance for the problem of m rankings. The An-
nals of Mathematical Statistics, 11:86–92.
Jiang, J., Zhang, J., Sun, J., Wu, D., and Xu, S. (2023).
User’s image perception improved strategy and appli-
cation of augmented reality systems in smart medical
care: A review. The International Journal of Medical
Robotics and Computer Assisted Surgery, 19.
Jud, L., Fotouhi, J., Andronic, O., Aichmair, A., Osgood,
G., Navab, N., and Farshad, M. (2020). Applicability
of augmented reality in orthopedic surgery – a system-
atic review. BMC Musculoskeletal Disorders, 21:103.
Li, L., Yang, J., Chu, Y., Wu, W., Xue, J., Liang, P.,
and Chen, L. (2016). A novel augmented real-
ity navigation system for endoscopic sinus and skull
base surgery: A feasibility study. PLOS ONE,
11:e0146996.
Mai, H.-N., Dam, V. V., and Lee, D.-H. (2023). Accuracy
of augmented reality–assisted navigation in dental im-
plant surgery: Systematic review and meta-analysis.
Journal of Medical Internet Research, 25:e42040.
Meola, A., Cutolo, F., Carbone, M., Cagnazzo, F., Fer-
rari, M., and Ferrari, V. (2017). Augmented reality
in neurosurgery: a systematic review. Neurosurgical
Review, 40:537–548.
Peh, S., Chatterjea, A., Pfarr, J., Sch
¨
afer, J. P., Weuster,
M., Kl
¨
uter, T., Seekamp, A., and Lippross, S. (2020).
Accuracy of augmented reality surgical navigation for
minimally invasive pedicle screw insertion in the tho-
racic and lumbar spine with a new tracking device.
The Spine Journal, 20:629–637.
Ruggiero, F., Cercenelli, L., Emiliani, N., Badiali, G.,
Bevini, M., Zucchelli, M., Marcelli, E., and Tarsitano,
A. (2023). Preclinical application of augmented re-
ality in pediatric craniofacial surgery: An accuracy
study. Journal of Clinical Medicine, 12:2693.
Scherl, C., Stratemeier, J., Karle, C., Rotter, N., Hesser, J.,
Huber, L., Dias, A., Hoffmann, O., Riffel, P., Schoen-
berg, S. O., Schell, A., Lammert, A., Affolter, A., and
M
¨
annle, D. (2021). Augmented reality with hololens
in parotid surgery: how to assess and to improve accu-
racy. European Archives of Oto-Rhino-Laryngology,
278:2473–2483.
Schiavina, R., Bianchi, L., Chessa, F., Barbaresi, U.,
Cercenelli, L., Lodi, S., Gaudiano, C., Bortolani,
B., Angiolini, A., Bianchi, F. M., Ercolino, A.,
Casablanca, C., Molinaroli, E., Porreca, A., Golfieri,
R., Diciotti, S., Marcelli, E., and Brunocilla, E.
(2021). Augmented reality to guide selective clamp-
ing and tumor dissection during robot-assisted par-
tial nephrectomy: A preliminary experience. Clinical
Genitourinary Cancer, 19:e149–e155.
Thabit, A., Benmahdjoub, M., van Veelen, M.-L. C.,
Niessen, W. J., Wolvius, E. B., and van Walsum, T.
(2022). Augmented reality navigation for minimally
invasive craniosynostosis surgery: a phantom study.
International Journal of Computer Assisted Radiology
and Surgery, 17:1453–1460.
van Doormaal, T. P. C., van Doormaal, J. A. M., and
Mensink, T. (2019). Clinical accuracy of holo-
graphic navigation using point-based registration on
augmented-reality glasses. Operative Neurosurgery,
17:588–593.
Verhellen, A., Elprama, S. A., Scheerlinck, T., Aerschot,
F. V., Duerinck, J., Gestel, F. V., Frantz, T., Jansen,
B., Vandemeulebroucke, J., and Jacobs, A. (2023).
Exploring technology acceptance of head-mounted
device-based augmented reality surgical navigation in
orthopaedic surgery. The International Journal of
Medical Robotics and Computer Assisted Surgery.
IVAPP 2024 - 15th International Conference on Information Visualization Theory and Applications
622