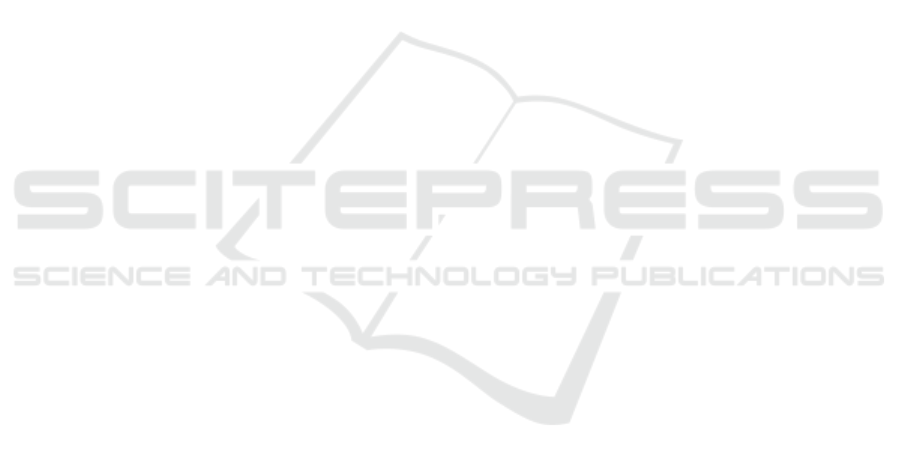
HUS (2023). HUSLAB - Natrium, vuorokausivirtsasta —
huslab.fi. https://huslab.fi/ohjekirja/2376.html.
[Accessed 02-11-2023].
Kordesch, K. and Taucher-Mautner, W. (2009). Primary
batteries – aqueous systems — leclanch
´
e and
zinc–carbon. In Garche, J., editor, Encyclopedia
of Electrochemical Power Sources, pages 43–54.
Elsevier, Amsterdam.
Lide, D. R., editor (2009). CRC handbook of chemistry and
physics, 90th edition. CRC Press, Boca Raton, FL, 90
edition.
Linden, D. and Reddy, T. (2001). Handbook of batteries.
Handbook Series. McGraw-Hill Professional, New
York, NY, 3 edition.
Moayer, M. M., Salomaa, J., and Halonen, K. A. I. (2020).
A 0.39–3.56-µW Wide-Dynamic-Range Universal
Multi-Sensor Interface Circuit. IEEE Sensors Journal,
20(20):12262–12273.
Muhthassim, B., Thian, X. K., and Hasan, K. N. M.
(2018). Energy harvesting from salinity gradient. IOP
Conference Series: Earth and Environmental Science,
140:012045.
Murtom
¨
aki, L., Kallio, T., Lahtinen, R., and Ikonen, M.
(2018). Fundamental electrochemistry: An interactive
e-book with quizzes in mycourses learning platform.
NJ-Gov (2016). nj.gov. https://www.nj.gov/health/eoh/rtkw
eb/documents/fs/0013.pdf. [Accessed 02-11-2023].
Pelner, L. (1956). TOXIC EFFECTS OF AMMONIUM
CHLORIDE. Journal of the American Medical
Association, 162(1):63.
Pulkkinen, M., Haapala, T., Salomaa, J., and Halonen, K.
(2020). Low-power wireless transceiver with 67-nW
differential pulse-position modulation transmitter.
IEEE Transactions on Circuits and Systems I: Regular
Papers, 67(12):5468–5481.
Pulkkinen, M., Salomaa, J., Moayer, M. M., Haapala,
T., and Halonen, K. (2017). 462-nW 2-axis gesture
sensor interface based on capacitively controlled ring
oscillators. In 2017 IEEE International Symposium on
Circuits and Systems (ISCAS). IEEE.
Putnam, D., Company-West, M. D. A., Center, L. R.,
Aeronautics, U. S. N., and Administration, S. (1971).
Composition and Concentrative Properties of Human
Urine. NASA contractor report. National Aeronautics
and Space Administration.
Rani, E. F., Elumalai, M., and Balasubramanian, M. P.
(1998). Water, Air, and Soil Pollution, 104(1/2):1–8.
Reports, W. M. (2022). Global Zinc Carbon
Battery Market – Market Reports
World — marketreportsworld.com. h t t p s :
//www.marketreportsworld.com/global- zinc- c
ar b o n- b at te r y- m ar ke t- 2 10 62 60 4. [Accessed
31-10-2023].
Singh, A. K., Pahlevaninezhad, M., Yasri, N., and Roberts,
E. P. L. (2021). Degradation of carbon electrodes in
the all-vanadium redox flow battery. ChemSusChem,
14(9):2100–2111.
Tanweer, M., Gillan, L., Sepponen, R., Tanzer, I. O.,
and Halonen, K. A. (2023a). Evaluation of printed
coplanar capacitive sensors for reliable quantification
of fluids in adult diaper. In MEDICON & CMBEBIH
2023. Springer International Publishing.
Tanweer, M., Monga, D. C., Gillan, L., Sepponen, R.,
Tanzer, I. O., and Halonen, K. A. (2023b). Smart
diaper with printed capacitive sensors and integrated
front-end to monitor voided fluid volume. IEEE
Sensors Journal [submitted 2023].
Tanweer, M., Sepponen, R., Tanzer, I. O., and Halonen,
K. A. (2023c). Development of capacitive sensors
to detect and quantify fluids in the adult diaper.
In Bio-inspired Information and Communications
Technologies, pages 237–245. Springer Nature
Switzerland.
Tools Store, T. L. o. (2022). 5 Stück
reines Zinkblech, hohe Reinheit 99,99
für wissenschaftliches Labor, 140 x
140 x 0,2 mm. : Amazon.de: Gewerbe,
Industrie & Wissenschaft — amazon.de.
https://www.amazon.de/dp/B096SDJS9K. [Accessed
06-11-2023].
BIODEVICES 2024 - 17th International Conference on Biomedical Electronics and Devices
70