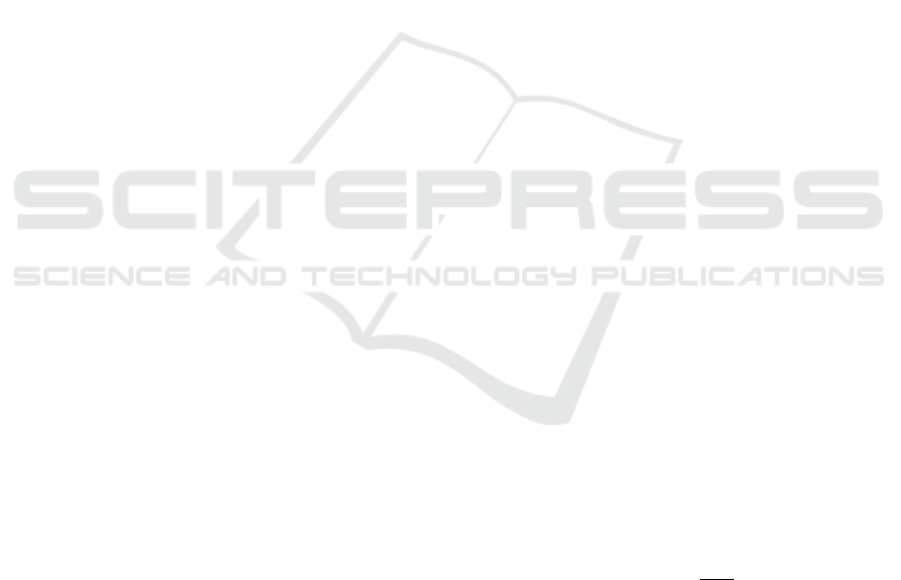
a gold nanorod array embedded in a Al
2
O
3
matrix
(Nicholls et al., 2017). Another possibility is repre-
sented by epsilon-near-zero materials-based devices,
such as in (Wang et al., 2021), where indium titanium
oxide is coupled to gold nanoantennas.
An alternative route is represented by meta-
surfaces, which are planar arrangements of tightly
packed nanoresonators, and, as such, are both ultra-
compact and highly tunable. Consequently, they con-
stitute the ideal candidate for the development of inte-
grated photonic devices. A relevant example of polar-
ization control is illustrated in a paper by some of the
present authors (Schirato et al., 2020), where a siz-
able dichroic response is induced in an isotropic plas-
monic metasurface via photo-induced excitation of
out-of-equilibrium carriers with a non-uniform spa-
tial pattern. In this sense, a complementary approach
consists in employing an anisotropic metasurface in
which pumping could induce a transient modulation
of static dichroism and birefringence (Della Valle
et al., 2017).
Here, following this last strategy, we present an
anisotropic AlGaAs metasurface, very efficiently re-
configurable by all-optical means, and showing gi-
ant modulations of its dichroic properties on an ul-
trafast timescale. We combine experiments with the-
oretical simulations, by performing ultrafast pump-
probe spectroscopy and multisteps modelling to ratio-
nalize the dynamics of the retrieved transient optical
response.
The paper is organized as follows. In section 2 the
design of the metasurface is described, and the results
of measurements are shown; an account of modelling,
as well as the discussion on experimental results, can
be found in section 3. Finally, in section 4, some con-
clusions are drawn about the presented picture, and
considerations about ongoing and future work are pre-
sented.
2 DESIGN AND EXPERIMENTS
The fabricated sample consists of two replicas of a
4 × 7 matrix of 70 µm × 70 µm AlGaAs nanowire
metasurfaces, each implementing different values of
the periodicity P and wires width W . The left panel
of figure 1 shows a sketch of one such metasurface,
as well as a pictorial depiction of the concept of ul-
trafast pump-probe spectroscopy. The left inset il-
lustrates the unit-cell vertical cross-section, while the
SEM image on the bottom right shows the top-view
of the horizontal xy−plane.
We report here measurements and results rela-
tive to the metasurface parameters P = 450 nm, W =
180 nm: this specific combination of parameters al-
lows to tune an extended resonance of the unper-
turbed structure in a spectral range comprised be-
tween 750 nm and 800 nm; such a range is of partic-
ular interest due to band-filling, which is the mecha-
nism presiding over the third-order optical nonlinear-
ity in semiconductors at the bandgap edge. Indeed,
in such region, the photoinduced permittivity modu-
lation following pump absorption is pronounced and
dominated by its real part; thus, nonlinear losses are
negliglible (Pogna et al., 2021). For the present case
of Al
0.2
Ga
0.8
As, the bandgap sits at around 745 nm.
The right panel of figure 1 shows the measured re-
flection spectrum of the unperturbed sample for light
polarized parallel (TE) or perpendicular (TM) with re-
spect to the nanowires (see inset), impinging at an an-
gle of approximately ∼ 10
◦
. The diameter of the spot-
size (FWHM) of the laser beam employed for probing
is approximately 250 µm, to be compared with the
diagonal of the metasurface (∼ 100 µm). Thus, the
sample is almost uniformly illuminated.
Note that the strong anisotropy of the metasurface
directly translates into a markedly dichroic static re-
sponse. A resonance in the TE polarization is located
at around 775 nm, whereas no resonant features are
observed upon illumination with TM polarized light.
It is also to be remarked that, due to probe spot-size
dimensions, the dominant contribution to the spec-
tra comes from light reflected by the substrate. In-
deed, we also measured the substrate optical response,
which, since an AlOx buffer is present, is configured
as the typical Fabry-Perot etalon response. In any
case, due to symmetry of quasi-normal incidence, the
substrate reflection spectra are almost degenerate in
the two different polarizations, and don’t show any
narrow resonant feature.
With the aim of characterizing the photo-induced
modulation of the dichroic properties of the meta-
surface, we performed ultrafast pump-probe spec-
troscopy measurements. These consist in illuminat-
ing the sample with a high intensity pump pulse and
then interrogating it with a second (lower intensity)
probe pulse, to record the relative variation of the
sample reflection, ∆R/R =
R
′
−R
R
, where R
′
ad R are
the reflection of the perturbed and unperturbed sam-
ple, respectively. This quantity is a function of both
the probe wavelength and the time delay between the
two pulses.
The experimental apparatus is constructed as fol-
lows: a Ti:sapphire laser that emits 100-fs pulses at
800 nm wavelength, with a repetition rate of 1kHz,
pumps a 1 mm Beta-Barium-Borate (BBO) crystal to
generate second harmonic pulses, centered at 400 nm.
These are directly employed as the optical pump in
NanoPlasMeta 2023 - Workshop on Nanophotonics, Plasmonics and Metamaterials
108