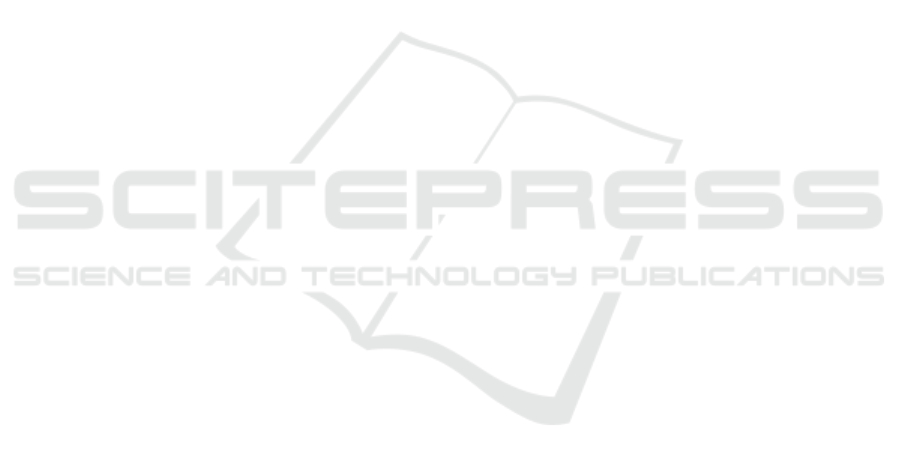
Lovett, P. B., Buchwald, J. M., St
¨
urmann, K., and Bijur, P.
(2005). The vexatious vital: Neither clinical measure-
ments by nurses nor an electronic monitor provides
accurate measurements of respiratory rate in triage.
Annals of Emergency Medicine, 45(1):68–76.
Marion, D. W. (2003). Therapeutic Moderate Hypothermia
and Fever. Cerebral Blood Flow, pages 272–276.
Massaroni, C., Nicol
`
o, A., Sacchetti, M., and Schena,
E. (2021). Contactless methods for measuring res-
piratory rate: A review. IEEE Sensors Journal,
21(11):12821–12839.
McKinlay, C. J., Chase, J. G., Dickson, J., Harris, D. L.,
Alsweiler, J. M., and Harding, J. E. (2017). Continu-
ous glucose monitoring in neonates: a review. Mater-
nal Health, Neonatology and Perinatology 2017 3:1,
3(1):1–9.
Miller, M. R., Hankinson, J., Brusasco, V., Burgos, F.,
Casaburi, R., Coates, A., Crapo, R., Enright, P.,
van der Grinten, C. P. M., Gustafsson, P., Jensen, R.,
Johnson, D. C., MacIntyre, N., McKay, R., Navajas,
D., Pedersen, O. F., Pellegrino, R., Viegi, G., and
Wanger, J. (2005). Standardisation of spirometry. Eu-
ropean Respiratory Journal, 26(2):319–338.
Moc¸o, A. and Verkruysse, W. (2021). Pulse oximetry based
on photoplethysmography imaging with red and green
light: Calibratability and challenges. Journal of Clin-
ical Monitoring and Computing, 35(1):123–133.
Mostafa, M., Hasanin, A. M., Elsayed, O. S., Mostafa,
M. M., and Sarhan, K. (2021). Accuracy of oscillo-
metric blood pressure measurement at both arms in
the lateral position. Blood Pressure Monitoring, pages
364–372.
Nedoma, J., Kepak, S., Fajkus, M., Cubik, J., Siska, P., Mar-
tinek, R., and Krupa, P. (2018). Magnetic Resonance
Imaging Compatible Non-Invasive Fibre-Optic Sen-
sors Based on the Bragg Gratings and Interferometers
in the Application of Monitoring Heart and Respira-
tion Rate of the Human Body: A Comparative Study.
Sensors 2018, Vol. 18, Page 3713, 18(11):3713.
Organization, W. H. (2022). Hypertension.
Pandian, P. S., Mohanavelu, K., Safeer, K. P., Kotresh,
T. M., Shakunthala, D. T., Gopal, P., and Padaki, V. C.
(2008). Smart Vest: Wearable multi-parameter remote
physiological monitoring system. Medical Engineer-
ing & Physics, 30(4):466–477.
Parak, J. and Havlik, J. (2011). ECG signal processing and
heart rate frequency detection methods. In Proceed-
ings of Technical Computing Prague.
Pham, M., Mengistu, Y., Do, H. M., and Sheng, W.
(2016). Cloud-Based Smart Home Environment
(CoSHE) for home healthcare. IEEE International
Conference on Automation Science and Engineering,
2016-November:483–488.
Pigini, L., Bovi, G., Panzarino, C., Gower, V., Ferratini,
M., Andreoni, G., Sassi, R., Rivolta, M. W., and Fer-
rarin, M. (2017). Pilot Test of a New Personal Health
System Integrating Environmental and Wearable Sen-
sors for Telemonitoring and Care of Elderly People at
Home (SMARTA Project). Gerontology, 63(3):281–
286.
Popescu, M. and Mahnot, A. (2012). Early illness recog-
nition using in-home monitoring sensors and mul-
tiple instance learning. Methods of information in
medicine, 51(4):359–367.
Rashidi, P. and Mihailidis, A. (2013). A survey on ambient-
assisted living tools for older adults. IEEE Journal of
Biomedical and Health Informatics, 17(3):579–590.
Rodr
´
ıguez, H., Williams, M., Wilkes, J. S., and Rogers,
R. D. (2008). Ionic liquids for liquid-in-glass ther-
mometers. Green Chemistry, 10(5):501–507.
Rose, L. and Clarke, S. P. (2010). Vital Signs. American
Journal of Nursing, 110(5):11.
Saquib, N., Papon, M. T. I., Ahmad, I., and Rahman,
A. (2015). Measurement of heart rate using pho-
toplethysmography. Proceedings of 2015 Interna-
tional Conference on Networking Systems and Secu-
rity, NSysS 2015.
Sardini, E. and Serpelloni, M. (2014). T-shirt for vital pa-
rameter monitoring. Lecture Notes in Electrical Engi-
neering, 162 LNEE:201–205.
Shivakumar, N. S. and Sasikala, M. (2014). Design of vital
sign monitor based on wireless sensor networks and
telemedicine technology. Proceeding of the IEEE In-
ternational Conference on Green Computing, Com-
munication and Electrical Engineering, ICGCCEE
2014.
Sixsmith, A., Johnson, N., and Whatmore, R. (2005). Pyro-
electric IR sensor arrays for fall detection in the older
population. Journal De Physique. IV : JP, 128:153–
160.
Spodick, D. H., Raju, P., Bishop, R. L., and Rifkin,
R. D. (1992). Operational definition of normal si-
nus heart rate. The American Journal of Cardiology,
69(14):1245–1246.
Subhi, R., Smith, K., and Duke, T. (2009). When should
oxygen be given to children at high altitude? A sys-
tematic review to define altitude-specific hypoxaemia.
Archives of Disease in Childhood, 94(1):6–10.
Sudana, D. and Emanuel, A. W. (2019). How Big Data
in Health 4.0 Helps Prevent the Spread of Tuberculo-
sis. Proceedings - 2019 2nd International Conference
on Bioinformatics, Biotechnology and Biomedical En-
gineering - Bioinformatics and Biomedical Engineer-
ing, BioMIC 2019.
Villar, R., Beltrame, T., and Hughson, R. L. (2015).
Validation of the Hexoskin wearable vest dur-
ing lying, sitting, standing, and walking ac-
tivities. https://doi.org/10.1139/apnm-2015-0140,
40(10):1019–1024.
Vinkers, C. H., Penning, R., Hellhammer, J., Verster, J. C.,
Klaessens, J. H., Olivier, B., and Kalkman, C. J.
(2013). The effect of stress on core and peripheral
body temperature in humans. The International Jour-
nal on the Biology of Stress, 16(5):520–530.
Wang, J. (2008). Electrochemical glucose biosensors.
Chemical Reviews, 108(2):814–825.
Monitoring of Vital Signs in the Home Environment: A Review of Current Technologies and Solutions
115