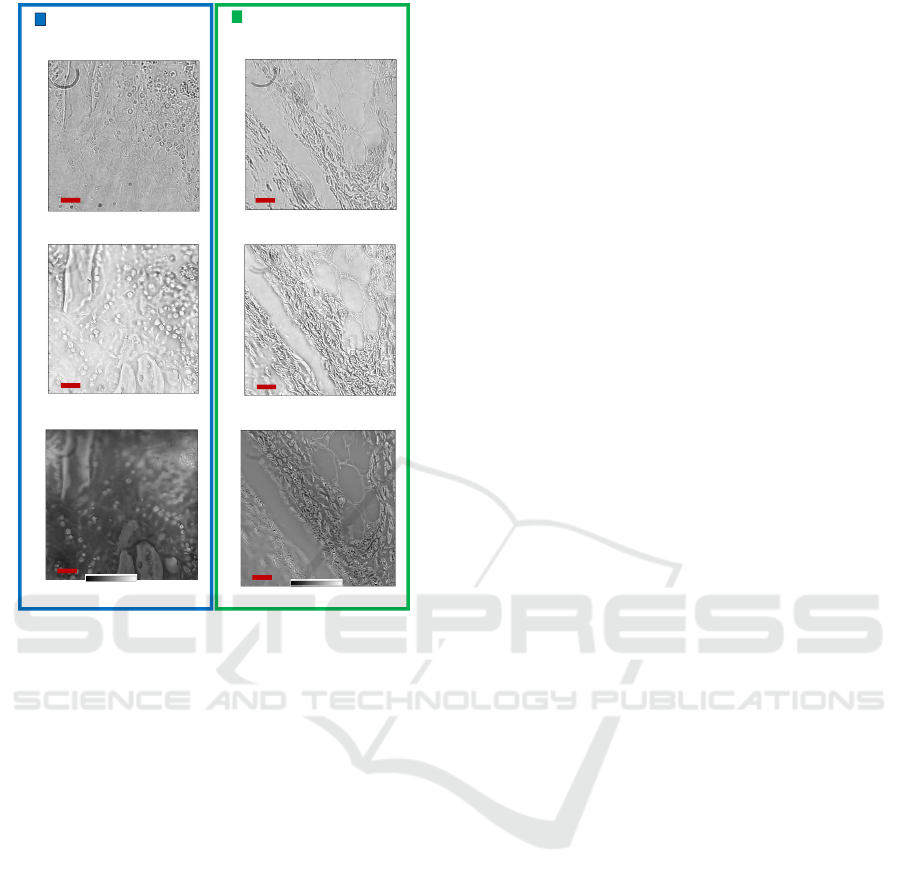
Low Resolution Image
Recovered Amplitude
Recovered Phase (rad)
λ = 530
nm
λ = 430
nm
Low Resolution Image
Recovered Amplitude
Recovered Phase (rad)
-𝜋
+𝜋
0
-𝜋/3
+𝜋
0
30 µm
30 µm
30 µm
30 µm
30 µm
30 µm
Figure 3: FPM amplitude and phase images of the cartilage
samples obtained with the green and blue LEDs.
nm. There is a good agreement between the measure-
ment and predicted theoretical values. Furthermore,
two histological cartilage samples were examined as
a practical example, showing a very fine details under
the achieved high NA
synth
by dome LED illuminator.
ACKNOWLEDGEMENTS
This work was supported by the Research Coun-
cil of Norway through NANO2021 (project number
272248), and FRIPRO (project number 275182).
REFERENCES
Eckert, R., Phillips, Z. F., and Waller, L. (2018). Efficient
illumination angle self-calibration in fourier ptychog-
raphy. Appl. Opt., 57(19):5434–5442.
Goodman, J. W. (2017). Introduction to Fourier optics.
W.H. Freeman Macmillan Learning, New York, fourth
edition. edition.
Konda, P. C. (2018). Multi-Aperture Fourier Ptychographic
Microscopy: development of a high-speed gigapixel
coherent computational microscope. PhD disserta-
tion, University of Glasgow.
Liu, Q., Kuang, C., Fang, Y., Xiu, P., Li, Y., Wen, R., and
Liu, X. (2016). Effect of spatial spectrum overlap on
fourier ptychographic microscopy. Journal of Innova-
tive Optical Health Sciences, 10.
Mayani, M. G., Breiby, D. W., and Akram, M. N.
(2022a). Polarization-sensitive Fourier ptychography
microscopy using dome-shaped LED illuminator. In
Georges, M. P., Popescu, G., and Verrier, N., editors,
Unconventional Optical Imaging III, volume 12136,
pages 31 – 34. International Society for Optics and
Photonics, SPIE.
Mayani, M. G., Hussain, N., Breiby, D. W., and Akram,
M. N. (Aug 2022b). Improved resolution fourier pty-
chography scheme using oil-filled dome-shaped LED
array. Electronics Letters, n/a(n/a).
Mico, V., Zalevsky, Z., Garc
´
ıa-Mart
´
ınez, P., and Garc
´
ıa, J.
(2006). Synthetic aperture superresolution with mul-
tiple off-axis holograms. Journal of the Optical Soci-
ety of America. A, Optics, image science, and vision,
23:3162.
Pan, A., Zhang, Y., Wen, K., Zhou, M., Min, J., Lei, M.,
and Yao, B. (2018). Subwavelength resolution fourier
ptychography with hemispherical digital condensers.
Opt. Express, 26(18):23119–23131.
Phillips, Z. F., D’Ambrosio, M. V., Tian, L., Rulison,
J. J., Patel, H. S., Sadras, N., Gande, A. V., Switz,
N. A., Fletcher, D. A., and Waller, L. (2015). Multi-
contrast imaging and digital refocusing on a mobile
microscope with a domed led array. PLOS ONE,
10:e0124938.
Phillips, Z. F., Eckert, R., and Waller, L. (2017). Quasi-
Dome: A Self-Calibrated High-NA LED Illuminator
for Fourier Ptychography. Imaging and Applied Op-
tics 2017, page IW4E.5.
Rimon, N. and Schuldiner, M. (2011). Getting the whole
picture: combining throughput with content in mi-
croscopy. Journal of Cell Science, 124(22):3743–
3751.
Tippie, A. E., Kumar, A., and Fienup, J. R. (2011). High-
resolution synthetic-aperture digital holography with
digital phase and pupil correction. Opt. Express,
19(13):12027–12038.
Zheng, G. (2016). Fourier Ptychographic Imaging. 2053-
2571. Morgan and Claypool Publishers.
Zheng, G., Horstmeyer, R., and Yang, C. (2013).
Wide-field, high-resolution Fourier ptychographic mi-
croscopy. Nature Photonics 2013 7:9, 7(9):739–745.
PHOTOPTICS 2023 - 11th International Conference on Photonics, Optics and Laser Technology
20