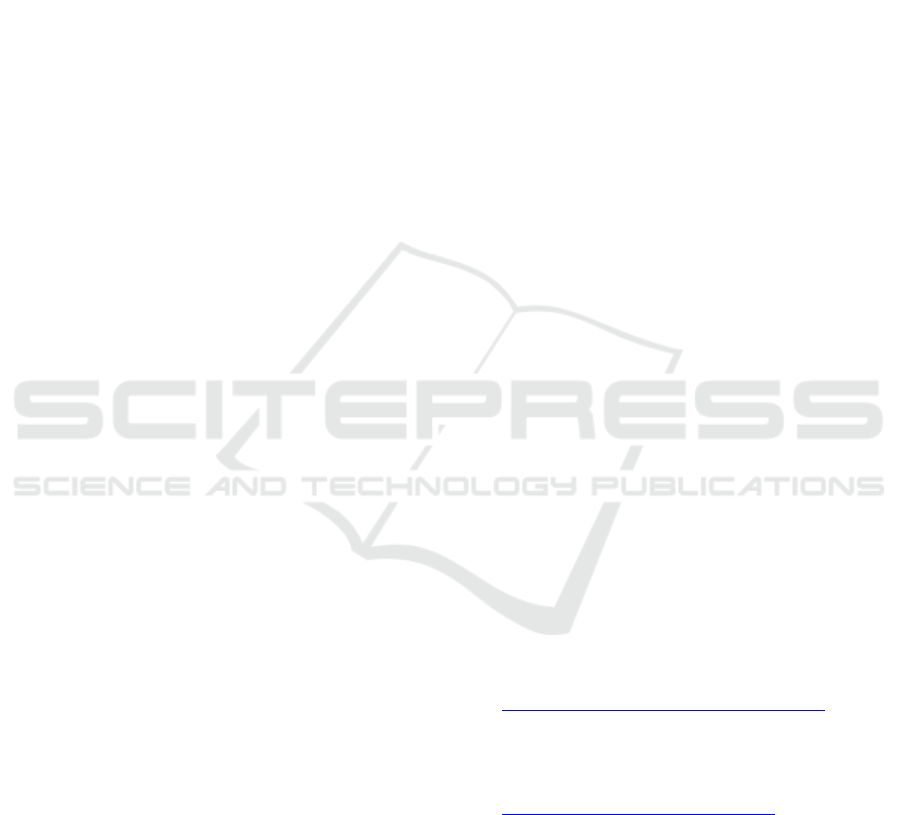
Chitosan nanoparticle and efficacy of miRNA
transfection
The MiR-155-5p expression is known to
experience downregulation in advanced stages of
ovarian cancer compared to early stages and
followed by upregulation of HIF1α mRNA
expression (Chasanah et al., 2016). Mimic-miRNA
is given to ovarian cancer cells to improve the
dysregulation of miR-155 5p on SKOV3.
The results of the agarose electrophoresis
inhibition test showed that mimic-miR 155-5p
trapped in irradiated chitosan (fig.2) to form
nanoparticles so that there is no free miRNA band.
This shows that nano complex can be formed
between mimic-miR-155-5p with irradiated chitosan
(Kaban et al., 2017; Martien, 2009; Wu et al., 2016).
The nanoparticle uptake test in SKOV3 cell
culture was carried out by transfecting mimic-
miRNA 155-5p nanoparticles for 2 different period,
4 hours (fig. 3b) and 48 hours (fig. 3c). The
irradiated chitosan-mimic miRNA 155-5p
nanoparticle transfection test was conducted by
observing whether there was an accumulation of
mimic-miRNA 155-5p green fluorescence around
the SKOV3 cell nucleus that had been stained with
blue dye DAPI. The nanoparticle formula showed
that at least 155-5p mimic-miRNA entered the cell
at the 4
th
hour of transfection and decreased at the
48
th
hour.
Particle size and charge from the surface of the
nanoparticles play an important role in the uptake of
nanoparticles to the cell and the efficiency of the
transfection system (Wu et al., 2016). Chitosan
irradiation could reduce the surface charge of the
particles due to amino group termination during the
radiation process. The results of FTIR showed the
bias of the absorption of amino groups showed in the
region 3300-3400 cm
-1
. This shows that during the
irradiation process there was an interruption of the
NH
3
+
group which caused the chitosan to lose its
positive charge.
4 CONCLUSIONS
5kGy gamma-rays irradiated chitosan showed a
reduction in molecular weight so that the viscosity
of chitosan solution was decrease than non-
irradiated chitosan. Irradiation indicates a break in
the main polysaccharides chain of chitosan. mimic-
miRNA 155-5p nanoparticle formulation with
irradiated chitosan showed good results in the
electrophoresis test but not in transfection test.
Irradiated chitosan nanoparticles were not able to
bring mimic-miRNA into the cell. This is possible
because the irradiation process cuts off the amino
group which makes the chitosan charge more
negative and is unable to carry mimic-miRNA 155-
5p through the positively charged cell membrane.
ACKNOWLEDGMENTS
The author thanks SCI KALBE, Center for
Application of Isotopes and Radiation, National
Nuclear Energy Agency, Indonesia and also LPPT
UGM as the opportunity to conduct the research.
REFERENCES
Chasanah, S. N., Fitriawan, A. S., Pukan, F. K., Kartika,
A. I., Oktriani, R., Trirahmanto, A., … Haryana, S. M.
(2016). The expression of miRNA-155 and hypoxia
inducible factor alpha (HIF1A) mRNA in the early
and advanced stages of ovarian cancer patients blood
plasma. Journal of Thee Medical Sciences (Berkala
Ilmu Kedokteran), 48(04 (Suplement)), 22–22.
https://doi.org/10.19106/JMedScieSup004804201620
Chen, Y., Wang, S.-X., Mu, R., Luo, X., Liu, Z.-S., Liang,
B., … Li, T. (2014). Dysregulation of the MiR-324-
5p-CUEDC2 Axis Leads to Macrophage Dysfunction
and Is Associated with Colon Cancer. Cell Reports,
7(6), 1982–1993.
https://doi.org/10.1016/j.celrep.2014.05.007
Czechowska-Biskup, R., Rokita, B., Ulanski, P., &
Rosiak, J. M. (2005). Radiation-induced and
sonochemical degradation of chitosan as a way to
increase its fat-binding capacity. Nuclear Instruments
and Methods in Physics Research Section B: Beam
Interactions with Materials and Atoms, 236(1–4),
383–390. https://doi.org/10.1016/j.nimb.2005.04.002
Desai, K. G., & Park, H. J. (2006). Study of Gamma-
Irradiation Effects on Chitosan Microparticles. Drug
Delivery, 13(1), 39–50.
https://doi.org/10.1080/10717540500309123
Kaban, K., Salva, E., & Akbuga, J. (2017). In Vitro Dose
Studies on Chitosan Nanoplexes for microRNA
Delivery in Breast Cancer Cells. Nucleic Acid
Therapeutics, 27(1), 45–55.
https://doi.org/10.1089/nat.2016.0633
Kinose, Y., Sawada, K., Nakamura, K., & Kimura, T.
(2014). The Role of MicroRNAs in Ovarian Cancer.
BioMed Research International, 2014, 1–11.
https://doi.org/10.1155/2014/249393
Martien, R. (2009). Oral Delivery of Nucleic Acid Drugs.
In A. Bernkop-Schnürch (Ed.), Oral Delivery of
Macromolecular Drugs (pp. 223–236).
https://doi.org/10.1007/978-1-4419-0200-9_12
Minagawa, T., Okamura, Y., Shigemasa, Y., Minami, S.,
& Okamoto, Y. (2007). Effects of molecular weight
Characterization and Prospect of Irradiated Chitosan as Nano Complex Material to Deliver MicroRNA in Cancer Therapy
195