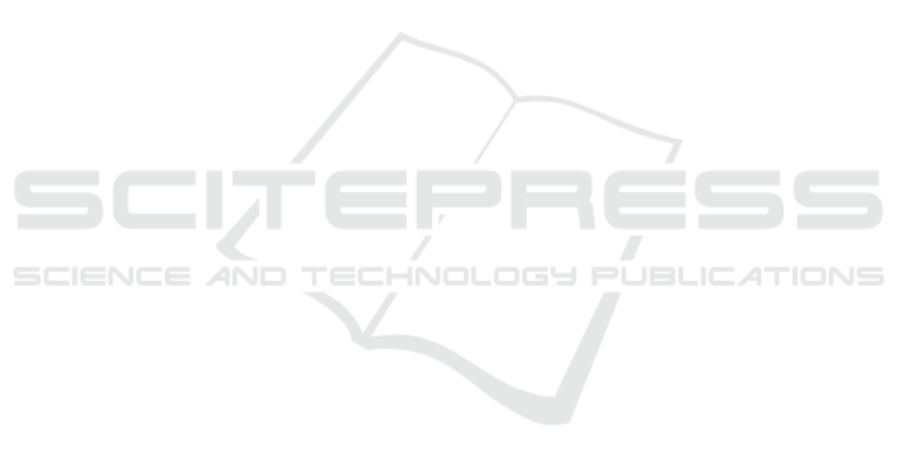
Bajpai, S. K., & Swarnkar, M. P. (2014). New Semi-IPN
Hydrogels Based on Cellulose for Biomedical
Application. Journal of Polymers, 2014, 1–12.
https://doi.org/10.1155/2014/376754
Bhattacharya, S. S., Mishra, A., Pal, D., Ghosh, A. K.,
Ghosh, A., Banerjee, S., & Sen, K. K. (2012).
Synthesis and Characterization of Poly (acrylic
acid)/Poly (vinyl alcohol)-xanthan Gum
Interpenetrating Network (IPN) Superabsorbent
Polymeric Composites. Polymer - Plastics Technology
and Engineering. https://doi.org/10.1080/
03602559.2012.671421
Brinchi, L., Cotana, F., Fortunati, E., & Kenny, J. M.
(2013). Production of nanocrystalline cellulose from
lignocellulosic biomass: Technology and applications.
Carbohydrate Polymers.
https://doi.org/10.1016/j.carbpol.2013.01.033
Burdick, J. A., & Stevens, M. M. (2005). Biomedical
hydrogels. Biomaterials, Artificial Organs and Tissue
Engineering, 107–115. https://doi.org/10.1533/
9781845690861.2.107
Chiellini, E., Corti, A., D’Antone, S., & Solaro, R. (2003).
Biodegradation of poly (vinyl alcohol) based
materials. Progress in Polymer Science (Oxford).
https://doi.org/10.1016/S0079-6700(02)00149-1
Ha, J., Kim, M., Lee, W., Lee, H., Han, C., Koh, W. G., &
Ryu, D. Y. (2018). Direct measurement of crosslinked
surface layer in superabsorbent poly (acrylic acid).
Materials Letters. https://doi.org/10.1016/j.
matlet.2018.05.079
Ioelovich, M., & Leykin, A. (2006). Microcrystalline
cellulose: Nano-structure formation. Cellulose
Chemistry and Technology.
Kiatkamjornwong, S. (2007). ScienceAsia, 33(s1), 039.
https://doi.org/10.2306/scienceasia1513-
1874.2007.33(s1).039
Mahdavinia, G. R., Zohuriaan-Mehr, M. J., & Pourjavadi,
A. (2004). Modified chitosan III, superabsorbency,
salt- and pH-sensitivity of smart ampholytic hydrogels
from chitosan-g-PAN. Polymers for Advanced
Technologies. https://doi.org/10.1002/pat.408
Maitra, J., & Shukla, V. K. (2014). Cross-linking in
Hydrogel - A Review. American Journal of Polymer
Science. https://doi.org/10.5923/j.ajps.20140402.01
Ni, J., Wen, Y., & Liu, H. (2015). Preparation of micro-
crystalline cellulose-reinforced polyacrylic acid
hydrogel and its application in paper/polyacrylic acid
composites. BioResources. https://doi.org/10.15376/
biores.10.3.4843-4853
Peresin, M. S., Habibi, Y., Vesterinen, A. H., Rojas, O. J.,
Pawlak, J. J., & Seppälä, J. V. (2010). Effect of
moisture on electrospun nanofiber composites of poly
(vinyl alcohol) and cellulose nanocrystals.
Biomacromolecules.
https://doi.org/10.1021/bm1006689
Saragih, G., Tamrin, T., Marpongahtun, Nasution, D. Y.,
& Abdillah. (2018). Preparation of semi-IPN hydrogel
from starch nanoparticles of magrove fruit and
monomer acrylic acid using crosslinker N,N’
methylene bisacrylamide. In AIP Conference
Proceedings. https://doi.org/10.1063/1.5082454
Sinha, S. (2018). Biodegradable superabsorbents: Methods
of preparation and application—A review.
Fundamental Biomaterials: Polymers. Elsevier Ltd.
https://doi.org/10.1016/B978-0-08-102194-1.00014-1
Spagnol, C., Rodrigues, F. H. A., Pereira, A. G. B.,
Fajardo, A. R., Rubira, A. F., & Muniz, E. C. (2012).
Superabsorbent hydrogel composite made of cellulose
nanofibrils and chitosan-graft-poly (acrylic acid).
Carbohydrate Polymers. https://doi.org/10.1016/j.
carbpol.2011.10.017
Sunardi, Irwan, A., Nurjannah, & Istikowati, W. T.
(2013). Pengaruh Penambahan Jumlah Inisiator
Amonium Persulfat (APS) Terhadap Karakteristik
Polimer Superabsorben Asam Akrilat dan Selulosa
Batang Alang-Alang (Imperata cylindrica). In
Posiding Seminar Nasional Penelitian, Pendidikan dan
Penerapan MIPA Fakultas MIPA, Universitas Negeri
Yogyakarta, ISBN: 978 – 979 -96880 – 7 - 1.
https://doi.org/10.13140/2.1.5170.2561
Swantomo, D., Megasari, K., & Saptaaji, R. (2008).
Pembuatan Komposit Polimer Superabsorben dengan
Mesin Berkas Elektron.
Teodorescu, M., Bercea, M., & Morariu, S. (2018).
Biomaterials of Poly (vinyl alcohol) and Natural
Polymers. Polymer Reviews. https://doi.org/10.1080/
15583724.2017.1403928
Vieira, R. P., Fernandes, A. R., Kaneko, T. M.,
Consiglieri, V. O., Pinto, C. A. S. D. O., Pereira, C. S.
C., … Velasco, M. V. R. (2009). Physical and
physicochemical stability evaluation of cosmetic
formulations containing soybean extract fermented by
Bifidobacterium animalis. Brazilian Journal of
Pharmaceutical Sciences.
https://doi.org/10.1590/S1984-82502009000300018
Yang, T. (2012). Mechanical and Swelling properties of
Hydrogels. Wiley.
ICOCSTI 2019 - International Conference on Chemical Science and Technology Innovation
318