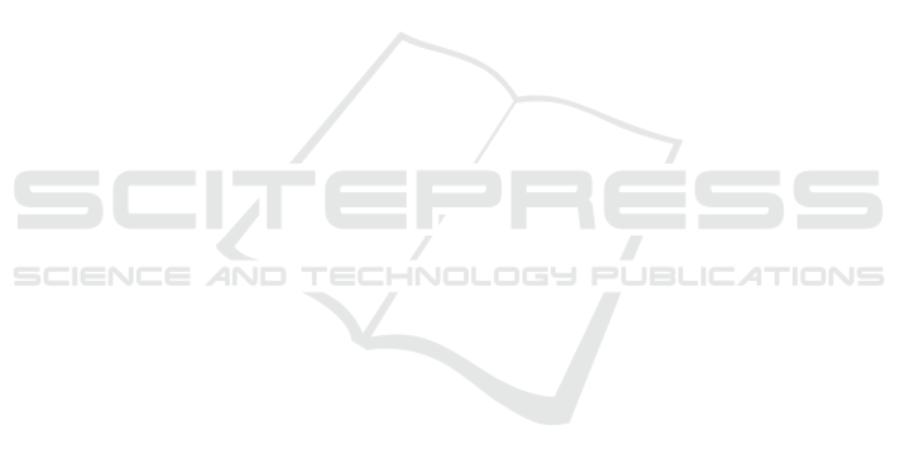
4 CONCLUSIONS
In this paper, the 3-D temperature distribution, cross
section temperature distribution, cross section
temperature gradient distribution of five-finger
power HBT with traditional emitter structure and
non-uniform emitter finger space structure at the
same dissipation power are given. Compared with 3-
D temperature distribution and cross section
temperature distribution, the difference between
traditional emitter structure and non-uniform emitter
finger space structure is more distinctive, so the
cross section temperature gradient distribution is
more effective for the design technique of non-
uniform finger spacing of multiple finger power
HBT.
REFERENCES
A Schuppen, U Erben, A Gruble. Enhanced SiGe HBT
with 160Hz-fmax[C]. IEEE International Electron
Devices Meeting Technical Digest, 1995: 743-746.
A Schuppen, S Gerlach, H Dietrich, et al. 1-W SiGe
Power HBT’s for Mobile Communication [J]. IEEE
Microwave Guided Wave Letters, 1996, 6(9): 341-343.
C Kermarrec, G Dawe, T Tewksbury. SiGe Technology:
Application to Wireless Digital Communications
[J].IEEE Microwave and Millimeter Wave Monolithic
Circuits Symposium. 1994: 1-4.
Jae-Sung Rieh,David Greenberg, Qizhi Liu, Alvin J.
Joseph, Greg Freeman, and David C. Ahlgren,
“Structure Optimization of Trench-Isolated SiGe
HBTs for Simultaneous Improvements in Thermal and
Electrical Performances,” IEEE Trans. Electron
Devices, vol. 52, no. 12, pp. 2744-2752, December
2005.
J. -S. Rieh, J. Johnson, S. Furkay, D. Greenberg, G.
Freeman, and S. Subbanna, “Structural Dependence of
the Thermal Resistance of Trench-Isolated Bipolar
Transistors,” IEEE BCTM, pp. 100-103, 2002.
P A Potyraj, K J Petrosky, K D Hobard. A 230-Watt SiGe
HBT [J]. IEEE Transactions on Microwave Theory
and Techniques, 1996, 44(12): 2392-2397.
Paul F. Combes, Jacques Graffeuil, Jean-Francois
Sautereau. Microwav components, devices and active
circuits. John Wiley & Sons, 1987, pp. 106.
S. P. McAlister, W. R. McKinnon, S. J. Kovacic, and H.
Lafontaine, “Self-heating in Multi-emitter SiGe HBTs,”
Solid-State Electronics, vol. 48, issues. 10-11, pp.
2001-2006, October-November 2004.
Wang Y, Zhang WR, Xie HY, and Zhang W, He LJ, Sha
YP, “Thermal analysis of power SiGe heterojunction
bipolar transistor with novel segmented multi-emitter
structure,” In: IEEE Proceedings of 2007 International
Conference on Microwave and Millimeter Wave
Technology, ICMMT '07. pp. 1–4.
Willian Liu, Ali Khatibzadeh, and Jim Sweder, “The Use
of Base Ballasting to Prevent the Collapse of Current
Gain in AlGaAs/GaAs HBTs,” IEEE Trans. Electron
Devices, vol. 43, no. 2, pp. 245-251, June 1996.
W. Liu, B. Bayraktaroglu, “Theoretical Calculation of
Temperature and Current Profiles in Multi-finger
HBTs,” Solid-State Electronics, vol. 36, no. 2, pp.
125-132, June. 1993.
A New Design Method for Emitter Finger Space of Heterojunction Bipolar Transistors
401