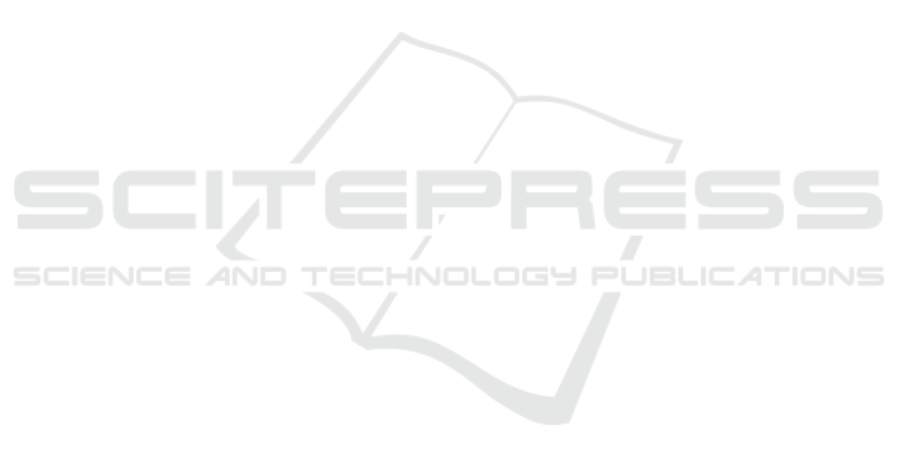
However, as the participant relaxes throughout the
duration of the run, they may move back down the
belt of the treadmill and must move forward again.
This motion requires a larger stride and greater
flexion and extension of the hip reasoning these large
peaks. The participants related this inconsistent pace
to inexperience running on a treadmill.
Peak changes in flexion and extension enable us
to understand the stage of each test the participant was
in when conducting the shuttle and agility drills. The
lower peaks suggest smaller and lighter steps
associated with changing direction and speed in the
shuttle runs, and this is evident in the breaks in the
peak accelerations (Figure 4). This occurred prior to
the larger peak flexion and extensions of the hip
associated with driving the knee forward to accelerate
quickly. This ability to sense a change in direction is
also notable in the agility tests (e.g. figure 14),
suggesting it may be possible to identify actions of
the athlete in a competitive scenario and hence
measure the performance metrics of the hip required
to perform such a movement. Opening the area of
competitive scenario research to identify
performance metrics associated with actions
performed in play, serving as an additional method of
performance evaluation. Such as the likes of the
capability of muscles about the hip to produce
moments when shooting in football, associating
muscular performance to speeds obtained by the ball
in flight. However, this will take a substantial amount
of time and case studies to support this hypothesis. As
well as substantial number of case studies to support
the correlation study of hip RoM and consequential
injures.
7 CONCLUSIONS
It was hypothesised that the measurement of RoM
and gait in a competitive scenario could identify the
position, motion and force through the hips and legs
prior to and at the time of injury. In doing so
supporting the real time injury analysis and the
diagnosis of injuries, by using motions at the hip and
their correlated driving muscles to identify possible
muscle damage and causes of pain and injury.
Both extra and intra articular injuries can be
identified by a change in the RoM at the hip.
However, large differences (-6.3º) in the sensor’s
readings, means that sound conclusions drawn as to
the exact angular position of the hip joint cannot be
made. However, it is possible to visualise the motion
of the upper leg. In cross examining video references
to the captured data, it is possible to identify
characteristics of an athlete’s form which may impact
performance. One such possible identification is from
the force measured through the leg in figure 10.
Showcasing participant 3’s fatigue over time with
lower peaks for turns eight and nine. Suspecting that
their muscles exhibited a lower force to decelerate as
they ran at a lower speed towards the end of the 10
repetitions. Such an example is relatively basic
however, showcases the desired foundations of
analysis of form and hip motion.
In testing on recreational athletes, it was possible
to differentiate form and gait characteristics in a
competitive scenario, unlike motion capture, giving a
closer insight into the demands of the lower limbs.
One such obvious example was the comparison of an
athlete’s acceleration and deceleration patterns.
Increased driving angle (Figure 10), cadence (Figure
5) and the forces exerted through the leg (Figure 5),
build a picture of the competitive performance of the
athletes. Whilst testing in this research is limited, the
findings are encouraging to show that a more detailed
analysis of the hip and the lower limbs is possible
when using our design. The shorts considerable lower
pricing point and ease of use make the design more
accessible to the general athletic market, laying the
foundations to better our understanding of the
competitive demands of the hip and lower limbs.
REFERENCES
Alonge, F. Cucco, E. D’Ippolito, F. Pulizzotto, A. (2014)
‘The Use of Accelerometers and Gyroscopes to
Estimate Hip and Knee Angles on Gait Analysis’,
Sensors (Basel), Vol.14(5), pp.8430-8446, [Online].
Available at: (Accessed: 3 March 2018)
Andersen, T. Larsen, O. Tenga, A. Engebretsen, L. Bahr, R.
(2003) ‘Football Incident Analysis: A New Video
Based Method to Describe Injury Mechanisms in
Professional Football’, British Journal of Sports
Medicine, Vol.37, pp.226-232, BMJ Journals, [Online].
Available at: https://bjsm.bmj.com/content/37/3/226?
ijkey=1228c77d7021287ddbe56a28d919e66d2d71aab
f&keytype2=tf_ipsecsha (Accessed: 16 November
2018)
Byrd, B. (2007) ‘Evaluation of the Hip: History and
Physical Examination’, International Journal of Sports
Physical Therapy, Vol.2, pp231-240, NCBI, [Online].
Available at: https://www.ncbi.nlm.nih.gov/pmc/
articles/PMC2953301/ (Accessed: 10 December 2017).
Elson, R. Aspinall, G. (2008) ‘Measurement of Hip Range
of Flexion-Extension and Straight-Leg Raising’,
Clinical Orthopaedics and Related Research,
Vol.466(2), pp.281,286, [Online] Available at:
https://www.ncbi.nlm.nih.gov/pmc/articles/PMC2505
147/ (Accessed: 17 March 2019).
icSPORTS 2019 - 7th International Conference on Sport Sciences Research and Technology Support
74