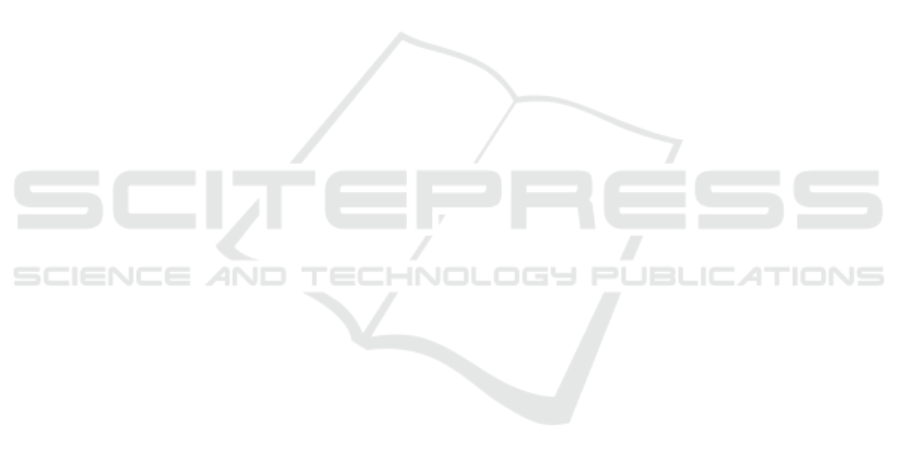
produce wash water. Two different filter designs
(vertical, gravity driven and rotating system) are
presented and tested. Adding multiple layers of ME
filter elements increases capture efficiencies.
Capture efficiencies of greater than 94% have been
demonstrated for flow velocity of 3mm/s.
Future research will address the effects of
temperature, flow rate, and water organic content on
capture efficiencies.
ACKNOWLEDGEMENTS
This material is based upon work, which is
supported by the National Institute of Food and
Agriculture (NIFA), U.S. Department of Agriculture
(USDA) and the Auburn University Detection Food
Safety Center (AUDFS).
REFERENCES
CDC, June 2018, https://www.cdc.gov/ecoli/
2018/o157h7-04-18/index.html.
CDC, December 2018, https://www.cdc.gov/ecoli/
2018/o157h7-11-18/index.html.
CDC, February 2018, https://www.cdc.gov/
foodsafety/foodborne-germs.html.
CDC, November 2018, https://www.cdc.gov/ salmonella/
index.html.
Chai Y, Li S, (2012) Rapid and sensitive detection of
Salmonella typhimurium on eggshells by using
wireless biosensors. Journal of Food Protection, 75
(4), 631-636.
Chai Y, Horikawa S, (2013a) A surface-scanning coil
detector for real-time, in-situ detection of bacteria on
fresh food surfaces, Biosens. Bioelectron, 50, 311.
Chai Y, Horikawa S, (2013b) Surface-scanning coil
detectors for magnetoelastic biosensors: A comparison
of planar-spiral and solenoid coils, Appl. Phys. Lett,
103, 173510.
Chai Y, Wikle H. C, (2013c) Design of a surface-scanning
coil detector for direct bacteria detection on food
surfaces using a magnetoelastic biosensor, J. Appl.
Phys, 114, 104504.
Guntupalli R, Lakshmanan R, (2007) Magnetoelastic
biosensor for the detection of Salmonella typhimurium
in food products, Sensing and Instrumentation for
Food Quality and Safety, Volume 1, Issue 1, Pages 3-
10. doi:10.1007/s11694-006-9003-8.
Guntupalli R, Sorokulova I, (2012) Detection and
identification of methicillin resistant and sensitive
strains of Staphylococcus aureus using tandem
measurements, J. Microbiol. Methods, 90(3), 182-91.
Huang S, Yang H, (2008a) The Effect of Salt and Phage
Concentrations on the Binding Sensitivity of
Magnetoelastic Biosensors for Bacillus anthracis
Detection, Biotechnol. Bioeng, 101, 1014-1021.
Huang S, Yang H, (2008b) Optimization of Phage-Based
Magnetoelastic Biosensor Performance, NSTI
nanotech 2008 conference, symposium on Phage
Nano-biotechnology, pp. 642-645.
Horikawa S, Chai Y, (2015) Direct detection of
Salmonella on fresh produce. ECS Transactions 69
(38), 25-31.
Li S, Horikawa S, (2012) Amorphous metallic glass
biosensors, Intermetallics 30, 80-85.
M. K. Park, J. W. Park, (2013a) Evaluation of phage-
based magnetoelastic biosensors by comparison with
TaqMan-based quantitative PCR, Sens. Actuators, B:
Chem, 176, 1134-1140.
Nambi S, Nyalamadugu S, (2003) Radio Frequency
Identification Sensors for Food Safety, Wireless
Communications Systems and Circuits Design in the
7
th
World Multi-Conference on Systemics, Cybernetics,
and Informatics, pp. 386-390.
Park M.K, Wikle H. C, (2012) The effect of incubation
time for Salmonella typhimurium binding to phage-
based magnetoelastic biosensors, Food Control,
Vol.26 (2), pp 539-545.
Park M. K, Li S, (2013b) Detection of Salmonella
typhimurium grown directly on tomato surface using
phage-based magnetoelastic biosensors, Food
Bioprocess Tech, 6(3), 682-689.
Staff, November 2018, FDA Update on Romaine Lettuce
Outbreak as Yuma, AZ, Growing Season Begins,
Food Safety Magazine.
Stephen Ostroff, M.D. June/July 2018. The Costs of
Foodborne illness, Product Recalls Make the Case for
Food Safety Investments. Food Safety Magazine.
Shen W, Mathison L.C, (2010) Design and
characterization of a magnetoelastic sensor for the
detection of biological agents, Journal of Physics D:
Applied Physics, 43 (2010) 015004. DOI:
10.1088/0022-3727/43/1/015004.
Biomolecular Phage Filter for the Detection of a Small Number of Pathogens in Large Volumes of Processing Water
113