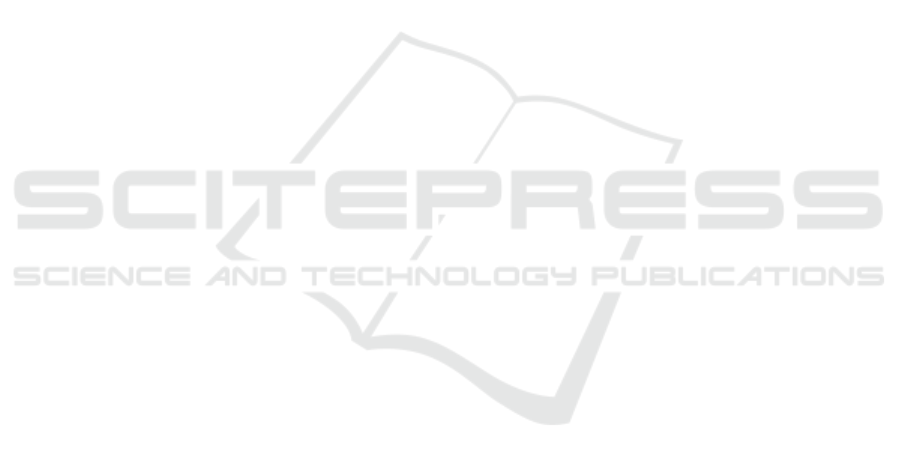
efficient electrons transport, and leading to a decrease of PCE as indicated by CdSe@TiO
2
HS solar
cells prepared by 36 h of hydrothermal process.
Figure 4(b) illustrated the working principle of CdSe@TiO
2
HS solar cells. The great potential to
use TiO
2
HS as supporting architecture in QDSSCs is that the hollow sphere structure not only
provides enough space for adsorption of QDs, but also generates an effect of full utilization of light
caused by multiple times reflection of light inside of hollow spheres as shown Figure 4 (b), leading to
the enhancement of light harvesting efficiency. When light is fully utilized to excite QDs generate
more electrons, the photovoltaic performance of QDSSCs can be improved. Therefore, our
CdSe@TiO
2
HS solar cell produced an acceptable PCE of 1.49%, showing great potential application
of TiO
2
hollow spheres in design of QDSSCs.
4. Conclusions
The TiO
2
hollow spheres were synthesized using carbonaceous spheres template method.
Furthermore, CdSe@TiO
2
hollow spheres photoanode were constructed by a simple hydrothermal
process using TGA as linker molecular. The TEM, SEM, and XRD analysis results proved our
strategy is feasible to obtain CdSe@TiO
2
hollow spheres photoanode for QDSSCs. The photovoltaic
performance analysis results showed that the hydrothermal reaction time can influence the I-V
behaviours of CdSe@TiO
2
hollow spheres solar cells. The QDSSCs with a better PCE of 1.49% can
be obtained by 24 h of hydrothermal reaction time. The proposed working principle of the solar cell
implies a potential application of TiO
2
hollow spheres for design of high power conversion efficiency
QDSSCs.
Acknowledgments
This work has been financially supported by the University Research Project of Gansu Province
[grant number 2017A-089], the Surface Project of Key Laboratory of Hexi Corridor Resources
Utilization of Gansu Province [grant number XZ1604], and the Hexi University Principle Fund of
Scientific Innovation and Application [grant number XZ2017010].
References
[1] Ren X, Yu L, Li Z, Song H and Wang Q 2018 Superlattices Microstruct. 113 696-705
[2] Lu Y B, Li L, Su S C, Chen Y J, Song Y L and Jiao S J 2017 RSC Adv. 7 9795-9802
[3] Li Z, Yu L, Liu Y and Sun S 2014 Electrochim. Acta 129 379-388
[4] Choi Y, Seol M, Kim W and Yong K 2014 J Phys. Chem. C. 118 5664-5670
[5] Cai Y, Wang H E, Zhao X, Huang F, Wang C, Deng Z, Li Y, Cao G and Su B L 2017 ACS
Appl Mater Interfaces 9 10652-10663
[6] Zhang Q, Chou T P, Russo B, Jenekhe S A and Cao G 2008 Angew Chem. Int. Ed. Engl. 47
2402-2406
[7] Lai X, Li J, Korgel B A, Dong Z, Li Z, Su F, Du J and Wang D 2011 Angew. Chem. Int. Ed.
Engl. 50 2738-2741
[8] Du J, Qi J, Wang D and Tang Z 2012 Energy & Environmental Science 5 6914
[9] Ren H, Yu R, Wang J, Jin Q, Yang M, Mao D, Kisailus D, Zhao H and Wang D 2014 Nano
Lett 14 6679-6684
[10] Wang Y, Shu Y, Xu J and Pang H 2017 Cryst Eng Comm 19 684-689
[11] Wang Y, Liu T, Huang Q, Wu C, Shan D and Mater J 2016 Res. 31 2317-2328
IWMCE 2018 - International Workshop on Materials, Chemistry and Engineering
518