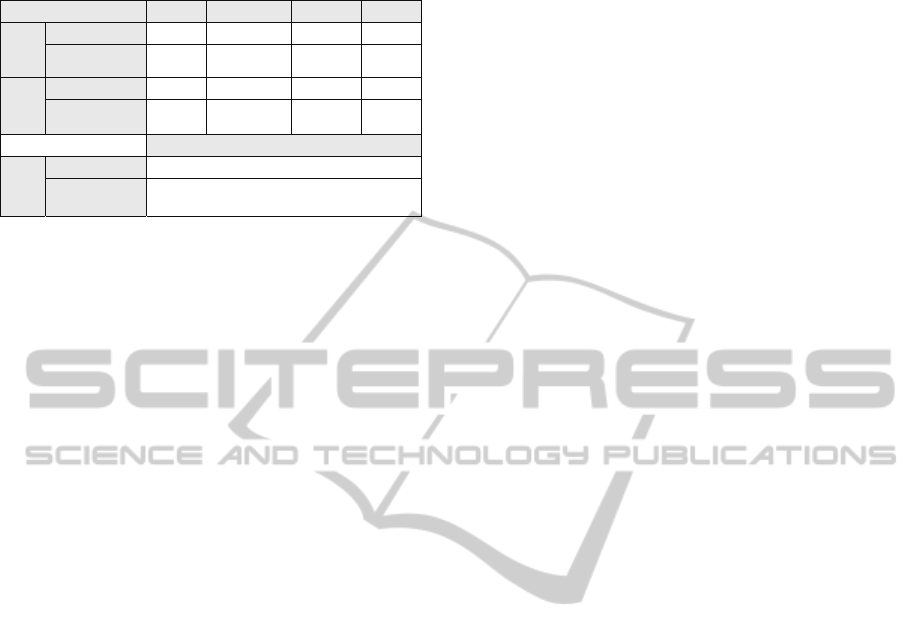
Table 4: Detail of the concordance test (m-a: mis-
assignment).
Group A B AB O
RCC
N° of tests
19 25 14 20
Concordance
%
100 100 100 100
WB
N° of tests
17 13 20 14
Concordance
%
100
84.6
2 m-a
95
1 m-a
94.4
1 m-a
All groups
RCC
+ WB
N° of test
142
Concordance
%
97
4 m-a
Mis-assignments reported here correspond to those
already mentioned in section 5.1.
6 CONCLUSIONS
In this paper, we presented a new device able to
semi-automatically perform an ultimate ABO
compatibility test. It is based on biochips grafted
with anti-A and anti-B antibodies. They are inserted
into disposable cartridges and placed into a mobile
and re-usable reader/actuator. The latter includes
embarked software that drives and controls the fluid
flows, performs the optical detection of captured red
cells and interpret the result in terms of ABO
compatibility.
In the current study, 292 biochips were tested.
The device exhibits sensitivity and specificity equal
to 99.3% and 97.9% respectively. We still need to
fully understand why 4
mis-assignments occurred
during these tests. However, for the 3 false positives,
washing was imperfect, probably due to a slight
motor dysfunction. For false negative biochips,
IgMs were probably not optimally grafted which
may explain the non-uniform red cells capture.
Optical reading and software interpretation are not to
be blamed. However, just after these 4 false results
have been observed, the same samples used with
new biochip were re-tested. This time, everything
worked correctly and no
mis-assignment was
observed.
Future work will consist in inserting the device
in the transfusion line and to control that RCC and
patient's blood are correctly driven onto the
biochips. Previous experiments (not shown here)
already demonstrated the efficient use of patient's
venous return in order to sample patient's blood with
minimum blood exposure risks.
To conclude, we believe that the
SmarTTransfuser concept may help enhancing blood
transfusion safety, not only in countries where a
double ultimate test is already performed, but also in
countries where only one test is considered.
Furthermore, such a device is meant to drastically
reduce non-compatibility accidents in countries
where the whole transfusion process (blood
donation, conservation, delivery and transfusion) is
not yet fully satisfactory.
ACKNOWLEDGEMENTS
This work was supported by the French
RENATECH network, the Etablissement Français
du Sang (EFS), the INSERM, the DGOS, the CNRS,
OSEO, the University of Franche-Comté
(“innovative project maturation” program) and the
European Community through the FEDER Program.
This work is developed in the frame of the Biom'@x
transversal axis at FEMTO-ST.
REFERENCES
ANSM, 2012 and 2014. Rapport d’activité hémovigilance.
http://ansm.sante.fr/Mediatheque/Publications/Bilans-
Rapports-d-activite-Bilans-et-rapports-d-
activite#folder_26762.
Berthier, A., Elie-Caille, C., Lesniewska, E., Delage-
Mourroux, R., Boireau, W., 2011. Label-free sensing
and atomic force spectroscopy for the characterization
of protein-DNA and protein-protein interactions:
application to estrogen receptors, J. Mol. Recognit.
JMR. 24, p.429–435.
Boozer, C., Kim, G., Cong, S., Guan, H., Londergan, T.,
2006. Looking towards label-free biomolecular
interaction analysis in a high-throughput format: a
review of new surface plasmon resonance
technologies, Curr. Opin. Biotechnol. 17, p. 400–405.
Campbell, C., Kim, G., 2007. SPR microscopy and its
applications to high-throughput analyses of
biomolecular binding events and their kinetics,
Biomaterials. 28, p. 2380–2392.
Charrière, K., Guerrini-Chapuis, J.S., Wacogne, B., Elie-
Caille, C., Pieralli, C., Pazart, L., Morel, P., Boireau,
W., 2011. SmartTransfuser: a lab-on-chip system for
enhancing transfusion security", 2
nd
International
Conference on Bio-sensing Technology, Amsterdam,
The Nederlands.
Charrière, K., Guerrini-Chapuis, J.S., Wacogne, B., Elie-
Caille, C., Pieralli, C., Pazart, L., Morel, P., Boireau,
W., 2012. SmarTTransfuser - A Biochip System for
the Final ABO Compatibility Test, in: SciTePress -
Science and and Technology Publications, Vilamoura,
p. 257-262.
Cid, J., Nogués, N., Montero, R., Hurtado, M., Briega, A.,
Parra, R., 2006. Comparison of three microtube
column agglutination systems for antibody screening:
BIODEVICES2015-InternationalConferenceonBiomedicalElectronicsandDevices
66