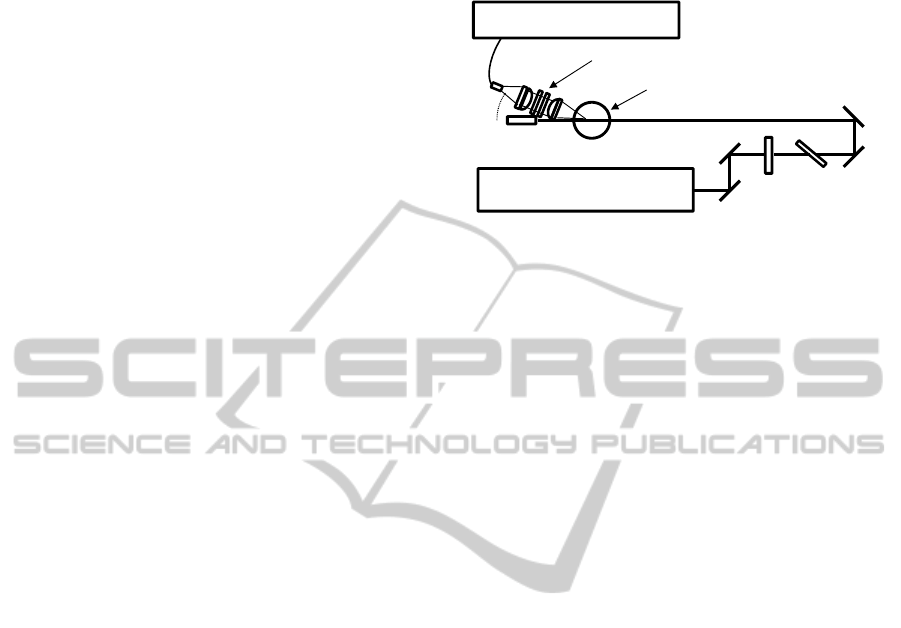
2 EXPERIMENTAL
2.1 Raman Spectroscopy
Figure 1 shows the schematic diagram of Raman
spectroscopy. The laser was a standard Q-switched
Nd:YAG laser (Continuum, Surelite: 10 ns pulse-
width with 100 mJ pulse energy at 10 Hz repetition
rate) operating at its second harmonic wavelength of
532 nm. A non-focused laser beam having about 8
mm diameter was used to avoid laser induced
damage of oil sample. The Raman signals from
samples are collected using an achromatic lens at an
angle of 25° from the forward direction of the laser
beam. This design provides a longer optical path
length than a conventional detection geometry at
90°, offering an order of magnitude increase in
Raman scattering intensity. After passing through
the edge and notch filters at 532 nm, the Raman
signal is coupled into an optical fiber bundle by
using an achromatic lens. The collected Raman
signal is dispersed by a spectrometer (Acton,
SpectraPro-2300i) with an entrance slit width set to
15 μm and detected with a liquid nitrogen-cooled
charge-coupled device (CCD) camera (Princeton
Instruments, SPEC-10). The exposure time was 90
ms. Accumulation numbers of C
2
H
2
and furfural
measurements were 3000 and 500, respectively.
Higher accumulation number in the C
2
H
2
detection
was required to reduce the random noise in Raman
spectra and improve the S/N ratio. The spectral
resolution of this system was estimated to be about 5
cm
-1
.
2.2 Sample Preparation
The insulating oil used in this work was a mixture of
naphthenic(41.6%), paraffinic(50.0%), and
aromatic(8.4%) oils. The used insulating oil
samples were optically clear in visible region. The
insulating oils were stored in glass bottles with
diameters of 3 cm. We confirmed that the glass
bottles had no effect on Raman spectra. After
complete degassing in vacuum for 4 hours, high-
purity C
2
H
2
gas (more than 99%) was introduced via
a gastight syringe. The C
2
H
2
concentrations of the
samples under the investigation were measured by
the gas chromatography and had 1.9%, 5.7%, and
10% concentrations, respectively.
Furfural is only slightly soluble in this oil.
Therefore, toluene solvent is added to oil. The
concentration of toluene in oil was constant at
approximately 9% for quantitative analysis. Furfural
used in this experiment becomes yellow on exposure
to air and light, but the spectrum obtained using 532
nm excitation is not dominated by fluorescence.
Edge+notchfilters
Surelite
(532nm,1W,10Hz)
Spectrometer+CCD
Oil,Furfural,To luene
25°
Polarizer
Half‐waveplate
M
M
M
M
Figure 1: Schematic diagram of the experimental setup.
3 RESULTS AND DISCUSSION
3.1 Raman Spectrum of Oil
Figure 2(a) shows the Raman spectra of oil. In short
and long edges of the spectrum, it exhibits numerous
features that are specific to complex oil structures
(Somekawa et al., 2013), however, it has no large
Raman spectral features and relatively low
background baseline between 1700 to 2500 cm
-1
range. The large peak centered at 1450 cm
-1
corresponds to CH
3
-CH
2
bending mode, and the set
of peaks at 1302 and 1350 cm
-1
corresponds to
paraffin C-H twisting modes. The peak at 1610 cm
-1
is due to an aromatic C=C stretching mode. The
band at 2725 cm
-1
can be assigned to the C-H
stretching mode. In higher wavenumber side not
shown here, the Raman spectrum of oil shows only
C-H stretching mode around 3000 cm
-1
, but no
signals in the region between 3100 and 4200 cm
-1
.
Figures 2(b) and 2(c) show the Raman spectra of
furfural and toluene, respectively, as discussed
below.
3.2 Raman Spectrum of C
2
H
2
Dissolved
in Oil
Figure 3 shows the spectra of C
2
H
2
gas at different
concentrations dissolved in the insulation oil. These
Raman spectra were normalized at ~2191 cm
-1
Raman signal intensity peaks. Weak Raman signals
were detected at 2191 cm
-1
, which were assigned to
the oil-derived Raman signal since its Raman peak
intensity remained almost unchanged as the C
2
H
2
concentration increased in the oil. On the other hand,
Raman peak intensity of relatively sharp line at
~1972 cm
-1
increased linearly versus increasing
C
2
H
2
concentration. We assign the peak around 1972
PHOTOPTICS2014-InternationalConferenceonPhotonics,OpticsandLaserTechnology
22