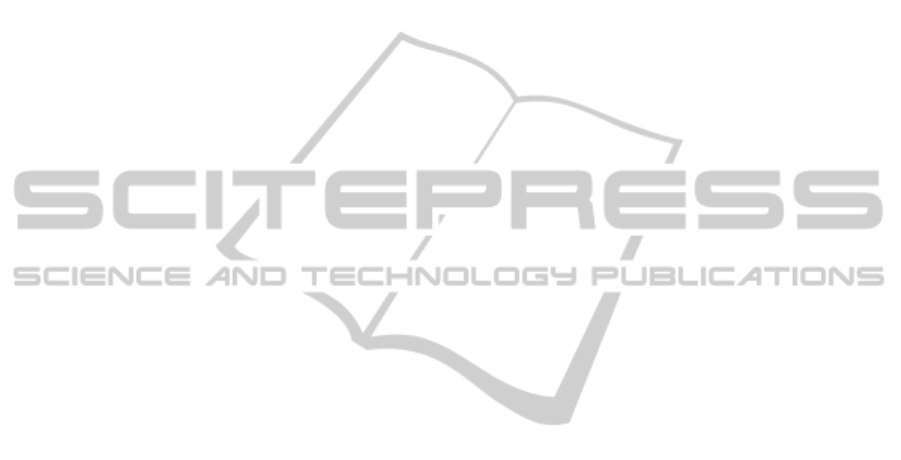
modular fashion with no lithography required, using
commercial valves and pumps when possible.
Printing, wet etching and print patterning have been
used to fabricate very inexpensive nanostructured
array chips. These devices can be set up in almost
any laboratory at a relatively low cost. However,
additional simplicity of operation is a goal for full
POC implementation. Our specific application is
detection of multiple proteins from serum and cell
lysates for cancer diagnostics, and this can be
achieved at detection limits down to 5 fg/mL
(attomolar levels), up to 200 times lower than
existing commercial multiplexed protein detection
systems (Rusling J. F., Kumar C. V., Gutkind J. S.,
et al. 2010).
ACKNOWLEDGEMENTS
This work is financially supported by grant
EB014586 from the National Institute of
Biomedical Imaging and Bioengineering (NIBIB),
NIH, USA.
REFERENCES
Chikkaveeraiah, B. V., Mani, V., Patel, V., Gutkind, J. S.,
Rusling. J. F. 2011. Microfluidic electrochemical
immunoarray for ultrasensitive detection of two cancer
biomarker proteins in serum, Biosensors &
Bioelectron. 26, 4477– 4483.
Giljohan D. A., Mirkin C. A. 2009. Drivers of
biodiagnostic development. Nature 462, 461-464.
Hanash S. M., Pitteri S. J., Faca V. M. 2008. Mining the
plasma proteome for cancer biomarkers. Nature 452,
571-579.
Hanash, S. M., Baik C. S., Kallioniemi, O. 2011.
Emerging molecular biomarkers—blood-based
strategies to detect and monitor cancer, Nature Rev.
Clin. Oncol. 8, 142–150.
Krause, C. E., Otieno, B. A., Latus, A., Faria R. C., Patel,
V., Gutkind, J. S., Rusling. J. F. 2013. Rapid
Microfluidic Immunoassays of Cancer Biomarker
Proteins using Disposable Inkjet-printed Gold
Nanoparticle Arrays, ChemistryOpen, 2, 141 – 145.
Kulasingam V, Diamandis E. P. 2008. Strategies for
discovering novel cancer biomarkers through
utilization of emerging technologies. Nature Clin.
Pract. Oncol. 5, 588-599.
Ludwig J. A., Weinstein J. N. 2005. Biomarkers in cancer
staging, prognosis and treatment selection. Nature
Rev. Cancer 5, 845-856.
Malhotra, R., Chikkaveeraiah, B. V., Patel, V. et al, 2012.
Oral Cancer Detection in the Clinic Using an
Ultrasensitive Microfluidic Array for a Panel of
Biomarker Proteins, Anal. Chem., 84, 6249–6255.
Manne U., Srivastava R. G., Srivastava S. 2005. Recent
advances in biomarkers for cancer diagnosis and
treatment. Drug Discov Today 10, 965-976.
Otieno, B. A., Krause, C. E., Latus, A., Chikkaveeraiah, B.
V., Faria R. C., Rusling. J. F. 2014. On-line Protein
Capture on Magnetic Beads for Ultrasensitive
Microfluidic Immunoassays of Cancer Biomarkers,
Biosens. & Bioelectron., 53, 268–274.
Rusling J. F., Kumar C. V., Gutkind J. S., et al. 2010.
Measurement of biomarker proteins for point-of-care
early detection and monitoring of cancer. Analyst 135,
2496–2511.
Rusling, J. F. 2012. Nanomaterials-based Electrochemical
Immunosensors for Proteins, Chem. Record, 12, 164–
176.
Rusling, J. F., Munge, B., Sardesai, N. P., Malhotra, R.,
Chikkaveeraiah, B. V. 2013. Nanoscience-based
electrochemical sensors and arrays for detection of
cancer biomarker proteins. Chapter 1, in Frank
Crespilho (Ed.), Nanobioelectrochemistry, Springer,
Berlin- Heidelberg, pp. 1-26.
Sardesai, N. P., Kadimisetty, K., Faria,
R., Rusling. J. F.,
2013. A microfluidic electrochemiluminescent device
for detecting cancer biomarker proteins, Anal.
Bioanal. Chem. 405, 3831-3838.
Tang, C. K., Vaze, A., Rusling. J. F. 2012. Fabrication of
immunosensor microwell arrays from gold compact
discs for ultrasensitive detection of cancer biomarker
proteins. Lab on a Chip, 12, 281-286.
BIODEVICES2014-InternationalConferenceonBiomedicalElectronicsandDevices
76