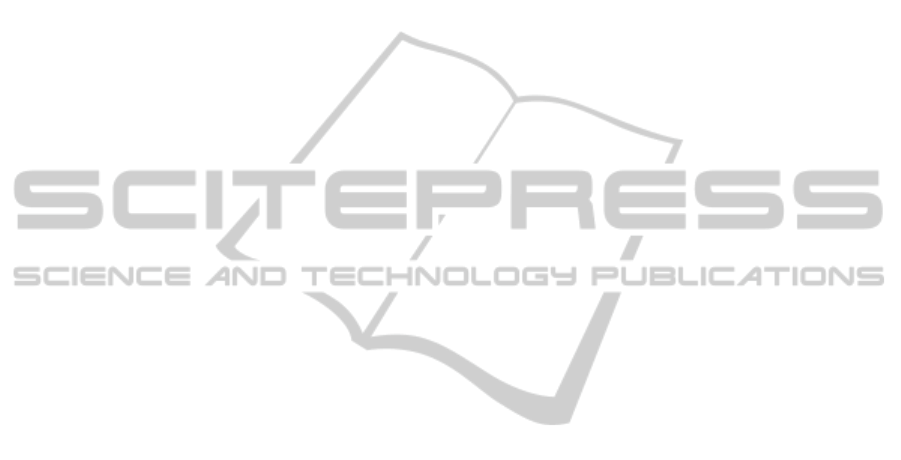
to trap cells prior to electrofusion. We demonstrated
the successful pairing and electrofusion between the
non-connected pads with biological cells. Thanks to
the absence of wiring, this method is a promising
technique for high density cell electrofusion on chip.
ACKNOWLEDGEMENTS
This work was performed thanks to the financial
support of PRES Universud, CNANO’Ile de France,
the ANR PNANO Nanopulsbiochip and the Labex
LASIPS, IMPcell project
REFERENCES
Köhler G. and Milstein C., 1975. Continuous cultures of
fused cells secreting antibody of predefined
specificity, In Nature, 256, 495.
Sukhorukov V. L., Reuss R., Endter J. M., Fehrmann S.,
Katsen-Globa A., Gessner P., Steinbach A., Müller K.
J., Karpas A., Zimmermann U. and Zimmermann H.,
2006. A biophysical approach to the optimisation of
dendritic-tumour cell electrofusion. In Biochemical
and Biophysical Research Communication, 346, 829.
Tada M., Takahama Y., Abe K., Nakatsuji N. and Tada T.,
2001. Nuclear reprogramming of somatic cells by in
vitro hybridization with ES cells. In Current Biology,
11, 1553.
Okada Y., 1958. The fusion of Ehrlich's tumor cells
caused by HVJ virus in vitro. In Biken Journal, 1, 103.
Pontecorvo G., 1975. Production of mammalian somatic
cell hybrids by means of polyethylene glycol
treatment. In Somatic Cell and Molecular Genetics, 1,
397.
Skelley A. M., Kirak O., Suh H., Jaenisch R. and Voldman
J., 2009. Microfluidic control of cell pairing and
fusion. In Nature Methods, 6, 147.
Zimmermann D., Terpitz
U., Zhou A., Reuss R., Müller
K., Sukhorukov V. L.,Gebner P., Nagel G.,
Zimmermann U. and Bamberg E., 2006. Biophysical
characterisation of electrofused giant HEK293-cells as
a novel electrophysiological expression system. In
Biochemical and Biophysical Research
Communications, 348 (2), 673.
Masuda S., Washizu M. and Nanba T., 1989. Novel
method of cell fusion in field constriction area in fluid
integrated circuit. In IEEE Trans. Ind. Appl, 25, 732,
1989
Techaumnat B., Tsuda K., Kurosawa O., Murat G., Oana
H. and Washizu M., 2008. High-yield electrofusion of
biological cells based on field tailoring by
microfabricated structures. In IET Nanobiotechnology,
2 (4), 93.
Kirschbaum M., Guernth-Marschner C. R., Cherré S., Pna
A., Jaeger M. S., Kroczek R. A., Schnelle T., Mueller
T. and Dusch C., 2012. Highly controlled
electrofusion of individually selected cells in
dielectrophoretic field cages. In Lab Chip, 12, 443
Dalmay C., Villemejane J., Joubert V, Francais O., Mir L.
M., Le Pioufle B., 2011. Design and realization of a
microfluidic device devoted to the application of ultra-
short pulses of electrical field to living cells. In
Sensors and Actuators B : Chemical, 160 (1),
1573Fricke H., 1924. J. of Applied Physics, 24, 575
Krupke R., Hennrich F., Kappes M. M. and Löhneysen
H., 2004. Surface Conductance Induced
Dielectrophoresis of Semiconducting Single-Walled
Carbon Nanotubes. In Nano Letters, 4 (8), 1395.
Salmanzadeh A., Kittu H., SSano M. B., Roberts P. C.,
Schmelz E. M., 2012. Dielectrophoretic differentiation
of mouse ovarian surface epithelial cells,
macrophages, and fibroblasts using contactless
dielectrophoresis. In Biomicrofluidics, 6, 024104.
BIODEVICES2013-InternationalConferenceonBiomedicalElectronicsandDevices
72