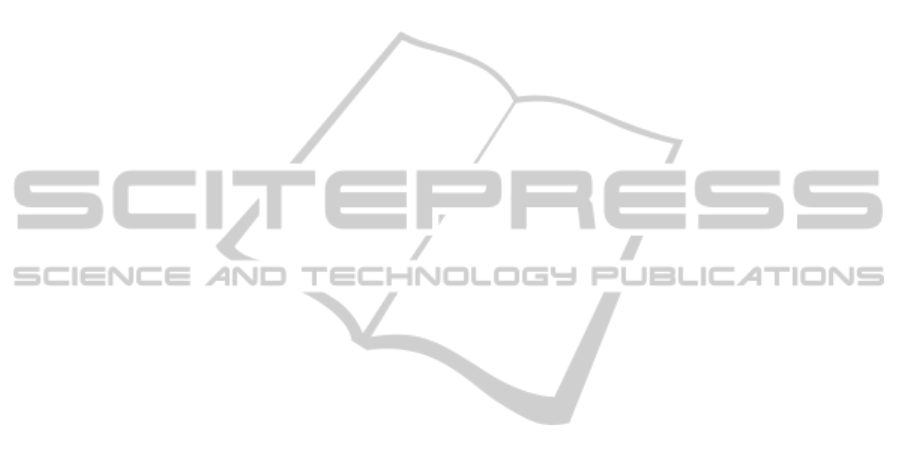
used as the disturbance (P_res-P_loads) in the smart
grids as in Fig.1.
The evaluation results are presented in Fig. 15.
One can see that the maximal power of the
renewable energy sources minus other loads is
130kW, but the maximal power of agent0 (through
transformer) is only 70kW. This evaluation results
prove that the architecture and principles (in
particular the virtual agent as presented in this
paper) can optimize (flatten) the average power flow
in the electric power distribution networks.
7 CONCLUSIONS
Market based operation in smart grids will ensure
fair transaction of electricity and enable more time
shift-able sources and loads involving in the
reconciliation of power imbalance. Particularly,
agent-based control and event-based control in smart
grids will change the characteristics of electric
power networks.
However, the power balance only between the
sources and the loads in agent-based control is not
sufficient to guarantee an appropriate power flow in
the electric power distribution networks. By
introducing a virtual agent, the principle of power
management in smart grids has been enhanced and
presented in this paper. The evaluation results show
that the average power flow in case of the
integration of renewable energy sources and
fluctuated loads can effectively be optimized by
applying our method of power management.
As there is a significant response time in demand
side management (via the ICT infrastructures), short
duration energy storage might be required, to ensure
the power quality in the smart grids, if the power
fluctuation due to the renewable energy sources (e.g.
PVs) or/and other fast varied loads is significant. In
this case, the possibly time shift-able and
controllable sources and loads of office building
(e.g. plug-in electric vehicles with super capacitors)
can be treated as another means of grid control. As a
result, power balance in smart grids can be achieved
in the different time scales with less additional
energy storages.
REFERENCES
J. M. Carrasco, L.G. Franquelo, J.T. Bialasiewicz, E.
Galvan, R.C. PortilloGuisado, M.A.M. Prats, J.I.
Leon, N. Moreno-Alfonso, "Power-Electronic Systems
for the Grid Integration of Renewable Energy Sources:
A Survey," IEEE Trans. on Industrial Electronics, vol.
53, no. 4, pp. 1002- 1016, August 2006.
Roman, R. Alonso, P. Ibanez, S. Elorduizapatarietxe, D.
Goitia, "Intelligent PV Module for Grid-Connected PV
Systems," IEEE Trans. on Industrial Electronics, vol.
53, no. 4, pp. 1066- 1073, August 2006.
E. Romero-Cadaval, M. I. Milanes-Montero, E. Gonzalez-
Romera, F. Barrero-Gonzalez, "Power Injection
System for Grid-Connected Photovoltaic Generation
Systems Based on Two Collaborative Voltage Source
Invert," IEEE Trans. on Industrial Electronics, vol. 56,
no. 11, pp. 4389-4398, Nov 2009.
A. Timbus, M. Larsson, C. Yuen, "Active Management of
Distributed Energy Resources Using Standardized
Communications and Modern Information
Technolog," IEEE Trans. on Industrial Electronics,
vol. 56, no. 10, pp. 4029-4037, Oct 2009.
Y. Cheng, Ph. Lataire, “New concepts for distributed
power generation and power quality for large scale
integration of renewable energy sources,” EPE2005, in
Dresden, Germany.
A. Y. Saber, G.K. Venayagamoorthy, "Plug-in Vehicles
and Renewable Energy Sources for Cost and Emission
Reductions ," IEEE Trans. on Industrial Electronics,
vol. 58, no. 4, pp. , April 2011.
Y. Cheng, “Methods for Mitigating the Effects of
Intermittent Energy Production of Photovoltaic
Sources,” the III International Conference on Power
Engineering, Energy and Electrical Drives (IEEE
POWERENG 2011), in Malaga, Spain, on 11 -13
May, 2011.
Y. Ueda, K. Kurokawa, T. Tanabe, K. Kitamura, H.
Sugihara, "Analysis Results of Output Power Loss
Due to the Grid Voltage Rise in Grid-Connected
Photovoltaic Power Generation Systems," IEEE Trans.
on IES, vol. 55, no. 7, pp. 2744-2751, July 2008.
J. Morren, S. W. H. de Haan, “Maximum penetration level
of distributed generation without violating voltage
limits,” CIRED 20th International Conference on
Electricity Distribution, June 2009.
Bletterie, T. Pfajfar, “Impact of Photovoltaic generation on
voltage variations -how stochastic is PV,” CIRED
19th International Conference on Electricity
Distribution, May 2007.
P. Souto Perez, J. Driesen, R. Belmans, “Characterization
of the Solar Power Impact in the Grid,” International
Conference on Clean Electrical Power, ICCEP '07.
2007.
Y. Cheng , “Power Management in Smart Grids for the
Integration of Renewable Energy Resources and
Fluctuated Loads,” ICCEP, 2011a, in Ischia, Italy.
Y. Cheng, “Fault-Tolerant Resonant Converters for Highly
Efficient and Reliable Power Conversion of Solar
Panels in Smart Grids,” the 14th International Power
Electronics and Motion Control Conference, (EPE-
PEMC2010), in Ohrid, Macedonia, on 6-8 September
2010.
V. C. Gungor, Bin Lu, G.P. Hancke, "Opportunities and
Challenges of Wireless Sensor Networks in Smart
SMARTGREENS2012-1stInternationalConferenceonSmartGridsandGreenITSystems
12