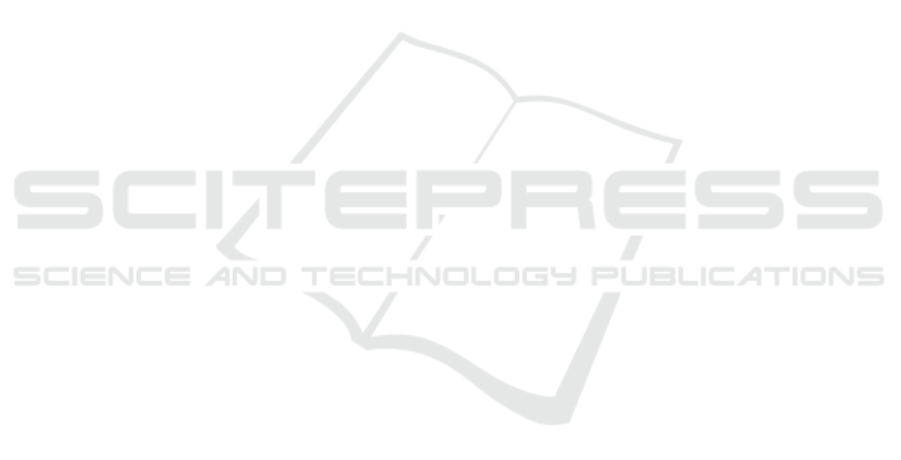
4 CONCLUSIONS
We demonstrated amplification 80 ns pulses to up to
20 kW of SBS free peak power (1.5 mJ). The
maximum peak power is limited by pump power
available in our experiment and father power scaling
using more powerful pump diode will be discussed
at the conference.
ACKNOWLEDGEMENTS
This work was supported with a grant 16-12-10553
from the Russian Science Foundation. Optimization
of MCVD fabrication process for achieving very flat
core refractive index was made within grant 16-03-
00325 of Russian Foundation for Basic Research.
Authors would like to thank A.A. Rybaltovsky and
O.V. Butov from Kotel’nikov Institute of Radio
Engineering and Electronics of RAS for provided
narrow-linewidth fiber Bragg gratings.
REFERENCES
Kotov, L. V. et al. (2016) ‘Monolithic high peak-power
coherent Doppler lidar system’, in Ballato, J. (ed.), p.
97282U.
Kotov, L. V et al. (2014) ‘Record-peak-power all-fiber
single-frequency 1550 nm laser’, Laser Physics
Letters, 11(9), p. 95102.
Jeong, Y. et al. (2005) ‘High-power tunable single-
frequency single-mode erbium:ytterbium codoped
large-core fiber master-oscillator power amplifier
source’, Optics Letters, 30(22), p. 2997.
Codemard, C. et al. (2006) ‘Millijoule, high-peak power,
narrow-linewidth, sub-hundred nanosecond pulsed
fibre Master-Oscillator Power-Amplifier at 1.55 µm’,
Comptes Rendus Physique, pp. 170–176.
Shi, W. et al. (2010) ‘Kilowatt-level stimulated-Brillouin-
scattering-threshold monolithic transform-limited 100
ns pulsed fiber laser at 1530 nm.’, Optics letters,
35(14), pp. 2418–20.
Kotov, L. V. et al. (2013) ‘75 W 40% efficiency single-
mode all-fiber erbium-doped laser cladding pumped at
976 nm’, Optics Letters, 38(13), p. 2230.
Jasapara, J. C. et al. (2009) ‘Diffraction-Limited
Fundamental Mode Operation Er Fiber Amplifiers’,
The IEEE Journal of Selected Topics in Quantum
Electronics, 15(1), pp. 3–11.
Canat, G. et al. (2013) ‘Kilowatt level transform-limited
150 ns monolithic pulsed fiber laser emitting in the L
band’, in 2013 Conference on Lasers and Electro-
Optics Europe and International Quantum Electronics
Conference, CLEO/Europe-IQEC 2013.
Peng, X. et al. (2013) ‘Root cause analysis and solution to
the degradation of wavelength division multiplexing (
WDM ) couplers in high power fiber amplifier
system’, Opt. Express, 21(17), pp. 20052–20061.
Jung, Y. et al. (2009) ‘Selective excitation of the
fundamental mode in a multimode fiber using an
adiabatically tapered splice’, in 2009 14th
OptoElectronics and Communications Conference.
IEEE, pp. 1–2.
Bobkov, K. et al. (2017) ‘Sub-MW peak power
diffraction-limited chirped-pulse monolithic Yb-doped
tapered fiber amplifier’, Optics Express, 25(22), pp.
26958–26972.
Kalaycioglu, H. et al. (2010) ‘Microjoule-energy, 1 MHz
repetition rate pulses from all-fiber-integrated
nonlinear chirped-pulse amplifier’, Optics Letters,
35(7), p. 959.
Kotov, L. et al. (2015) ‘Millijoule pulse energy 100-
nanosecond Er-doped fiber laser’, Optics Letters,
40(7), p. 1189.
Bogatyrev, V. A. et al. (1991) ‘A Single-Mode Fiber with
Chromatic Dispersion Varying Along the Length’,
Journal of Lightwave Technology, 9(5), pp. 561–566.
Bogatyrjov, V. A. and Sysoliatin, A. A. (2001) ‘An
efficient method to produce fibers with outer diameter
varying along the length’, Fiber Optic Sensor
Technology Ii, 4204, pp. 274–277.
High Peak Power Er-doped Tapered Fiber Amplifier
109