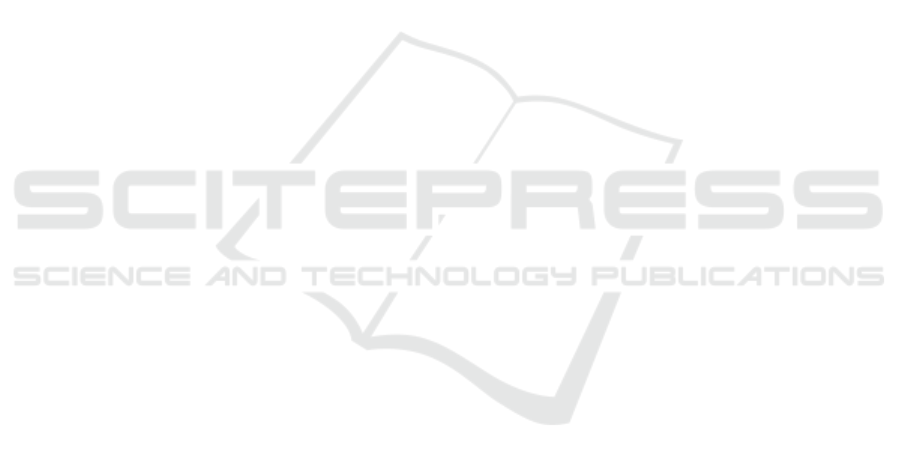
Photonics Defined Radio
A New Paradigm for Future Mobile Communication of B5G/6G
Zong Baiqing
1
, Zhang Xiaohong
1
, Li Xiaotong
1
, Wang Jianli
1
and Zhang Senlin
2
1
R&D Center (Shanghai), ZTE Corporation, Zhangjiang High-Tech Park, Shanghai, China
2
Global Marketing and Solution, ZTE UK Ltd, London, U.K.
Keywords: Fibre Optics Links and Subsystems, Radio Frequency Photonics, Photonic Integrated Circuits, Coherent
Communications, Optical Signal Processing, Radio Access Network, Beyond 5G.
Abstract: Photonics defined radio, a new and possibly standardized paradigm, is proposed, converging integrated
coherent optics, integrated microwave photonics and photonic DSP, and expected to dominate the designs
of future communication and sensing systems. The applications of photonics defined radio are also
discussed in the next generation cloud-based radio access network (CloudRAN), sensing and
communication integrated system, as well as artificial intelligence radio.
1 INTRODUCTION
Optics and photonics have made many progresses in
the past years, especially in the areas of integrated
coherent optics, integrated microwave photonics,
optical frequency comb and photonic digital signal
processing.
In 2006, Infinera reported the first
commercially deployed and indium phosphide (InP)
based large-scale photonic integrated circuits (LS-
PICs) of coherent optics (Jacco Pleumeekers et al,
2006). This breakthrough brought optical coherent
transmission back. Nowadays, coherent optical
transmission follows the trend migrating from long
haul market into metro and access arena with main
applications in mobile fronthaul of 5G/B5G. To use
linear phase modulation (PM) and coherent
detection (CD), high linear RF photonic or coherent
radio over fibre (CRoF) links have extensively been
realized. In particular, to employ a novel attenuation
counter-propagating optical phase-locked loop
(ACP-OPLL) demodulator the ultra-high linear
CRoF links have been obtained, and to use a hybrid
integrated or a monolithically integrated ACP-
OPLL, 140dB/Hz
2/3
spurious free dynamic range
(SFDR) over 1GHz bandwidth has been expected (S.
Jin et al, 2014). As a result, CRoF shows a
promising potential to future true cloud-based
5G/B5G applications.
Integrated microwave photonics (IMWP) has
become the most active area of current research and
development in microwave photonics. Based upon
various material platforms such as indium phosphide
(InP), silicon on insulator (SOI), Si photonics and
silicon nitride (Si3N4), several particular
functionalities and sub-systems have be realized
(David Marpaung et al, 2014), including tunable
filtering, optoelectronic oscillation, true time delay,
frequency up and down conversion, etc. In the mode
of monolithic or hybrid photonic circuits, IMWP is
evolving towards the so-called application specific
photonic integrated circuits (ASPICs) where a
particular circuit and chip configuration are designed
to optimally perform a particular functionality.
Furthermore, a photonic system-on-chip (PSoC) is
being developed, such as EU HAMLET project
which is the joint development of a new kind of
photonic frontend for future 5G/B5G mobile
networks in the frequency range of 28GHz, and
using hybrid photonic integration technology to
interface seamlessly the optical fronthaul and radio
access of the remote antenna units (RAU)
(http://www.ict-hamlet.eu/).
Coherent light source with wider spectrum is
critical to IMWP, CRoF and photonic digital signal
processing (DSP). Optical frequency comb (OFC) is
a revolutionary advance in broadband coherent
source. However, the bulk OFCs based on existing
mode-locked lasers have limited applications in real-
world due to their size, weight, power and cost.
Electro-optic or parametric frequency combs such as
Kerr micro-combs have later evolved based upon
Baiqing, Z., Xiaohong, Z., Xiaotong, L., Jianli, W. and Senlin, Z.
Photonics Defined Radio - A New Paradigm for Future Mobile Communication of B5G/6G.
DOI: 10.5220/0006551501550159
In Proceedings of the 6th International Conference on Photonics, Optics and Laser Technology (PHOTOPTICS 2018), pages 155-159
ISBN: 978-989-758-286-8
Copyright © 2018 by SCITEPRESS – Science and Technology Publications, Lda. All rights reserved
155