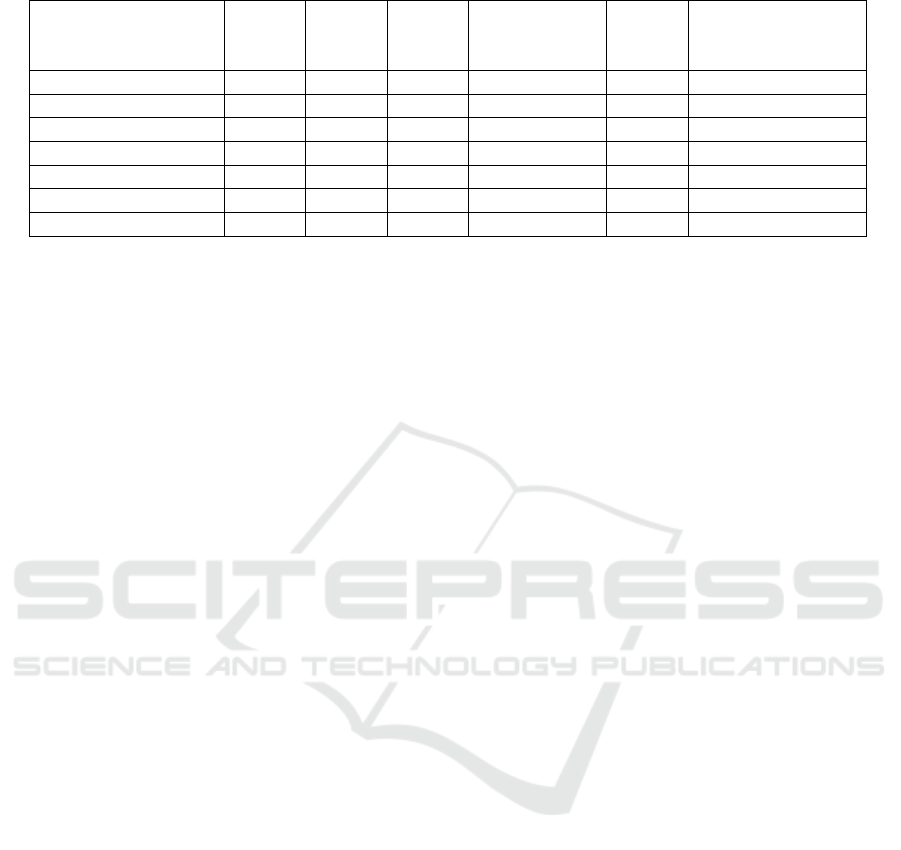
Table 3: State of the art rectifier output voltage comparison.
Architecture
Number
of
Diodes
Load
(kΩ)
P
in
(dBm)
Frequency
(MHz)
V
out
(mV)
Ref.
Full rectifier 2 8.2 -20 890 114 (Ho et al., 2016)
Full rectifier 2 5 -20 2450 <200 (Selim et al., 2020)
Full rectifier 4 NA NA 2450 50 (Chen et al., 2017)
Single ended rectifier 1 Inf -20 2450 150 This work
Single ended rectifier 1 Inf -8 2450 542 This work
Dickson 4 NA -8 2450 500 (Fan et al., 2018)
Full rectifier 2 5 -8 2450 <500 (Selim et al., 2020)
voltage (V
out
) of our rectifier for an incident power
(P
in
) of -8 dBm has been performed.
This table shows some voltage values (V
out
) ob-
tained with different rectifier configurations. We
can see that our single-ended rectifier obtains simi-
lar performance in terms of voltage as more complex
schematics. This is due to the use of a super capaci-
tor as a load that allows us to consider an infinite load
and therefore to obtain a better voltage.
We could see that our system allowed us to trans-
mit data from a connected object, which is battery
free and powered thanks to an RF energy harvesting
at 2.45 GHz. The association of our rectifier with a
voltage DC/DC boost and storage capacitors is able
to supply a voltage of 3.3 V with enough energy to
allow the sensor data transmission. Whether at 25,
50 or even 100 cm Tx/Rx distance, we can expect at
least, 4 transmissions per day.
We can then imagine application cases such as
temperature monitoring in offices in order to optimize
the heating of the building and thus hope to save fossil
fuels.
However, currently, this has only been possible
with an external system that manages the switches al-
lowing passing to one side or the other of the system
without losing energy. In-depth study work is nec-
essary on these switches to have a fully autonomous
device.
It will also be necessary to test this system not
with a constant power RF signal generator but with
a WiFi signal to determine a minimum network activ-
ity to allow transmission in a reasonable time.
REFERENCES
(2017). UB20M High-Voltage, Low-Threshold, Ultra-Low
Power Voltage Detector for Energy Harvesting, RF
Power Transfer, and Event-Driven Sensing. Bristol
Energy. Rev 2.2.
ADEME (2019). Mod
´
elisation et
´
evaluation environ-
nementale de produits de consommation et biens
d’
´
equipement.
Archos (2021). Archos and ossia partner to bring inovative
wirelessly powered consumer iot products to market.
Balguvhar, S. and Bhalla, S. (2018). Green Energy Harvest-
ing Using Piezoelectric Materials from Bridge Vibra-
tions. In 2018 2nd International Conference on Green
Energy and Applications (ICGEA), pages 134–137.
Berg
`
es, R., Fadel, L., Oyhenart, L., Vigneras, V., and Taris,
T. (2015). A dual band 915MHz/2.44GHz RF energy
harvester. In 2015 European Microwave Conference
(EuMC), pages 307–310. ISSN: null.
Chen, X., Huang, L., Xing, J., Shi, Z., and Xie, Z.
(2017). Energy harvesting system and circuits for am-
bient WiFi energy harvesting. In 2017 12th Interna-
tional Conference on Computer Science and Educa-
tion (ICCSE), pages 769–772. ISSN: 2473-9464.
Commission, E. (2020). Heating and cooling.
Correa-Betanzo, C., Lopez-Perez, C., Rodriguez, A., and
Lopez-Nu
˜
nez, A. (2019). Isolated DC-DC Con-
verter for Thermoelectric Energy Harvesting Based
on a Piezoelectric Transformer. In 2019 IEEE Ap-
plied Power Electronics Conference and Exposition
(APEC), pages 3443–3447.
ETSI (2019). Etsi en 300 328 v2.2.2 (2019-07).
Fan, S., Zhao, Y., Gou, W., Song, C., Huang, Y., Zhou,
J., and Geng, L. (2018). A high-efficiency radio fre-
quency rectifier-booster regulator for ambient WLAN
energy harvesting applications. In 2018 IEEE MTT-S
International Wireless Symposium (IWS), pages 1–3.
Franciscatto, B. R., Freitas, V., Duchamp, J.-M., Defay, C.,
and Vuong, T. P. (2013). High-efficiency rectifier cir-
cuit at 2.45 GHz for low-input-power RF energy har-
vesting. In 2013 European Microwave Conference,
pages 507–510. ISSN: null.
Grante, F., Abib, G. I., Muller, M., and Samama, N. (2020).
Autonomous sensor node powered over WiFi. In WIN-
SYS 2020: 17th International Conference on Wireless
Networks and Mobile Systems, volume 1: WINSYS,
pages 127–132, Lieusaint, France. ScitePress.
Ho, D.-K., Kharrat, I., Ngo, V.-D., Vuong, T.-P., Nguyen,
Q.-C., and Le, M.-T. (2016). Dual-band rectenna for
ambient RF energy harvesting at GSM 900 MHz and
1800 MHz. In 2016 IEEE International Conference
on Sustainable Energy Technologies (ICSET), pages
306–310. ISSN: null.
WINSYS 2022 - 19th International Conference on Wireless Networks and Mobile Systems
20