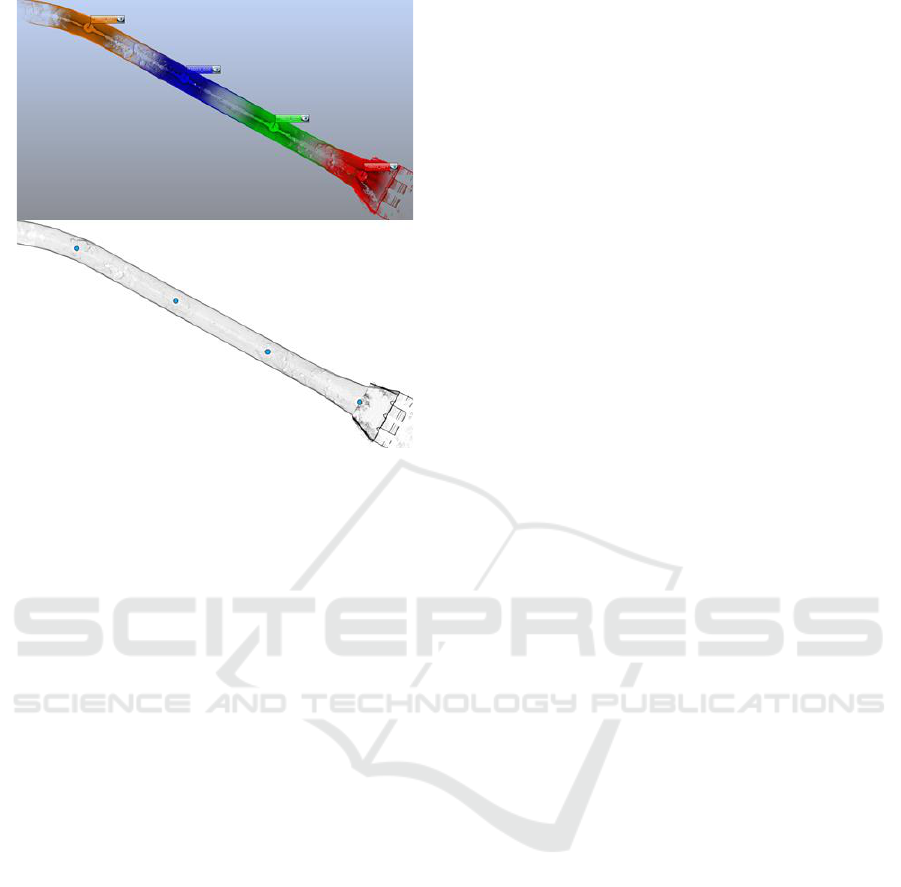
Figure 17: Point cloud of tufa tunnel section with four scan
location.
5 CONCLUSIONS
In this paper, we presented a new methodological
framework for analyzing (TFD) at three levels of
research using state-of-art active and passive sensors.
The 3D models of the first (reference)
measurement are presented fot three levels of
research. Although the next measurements will be
made in a year, interesting occurrences have already
been noticed. Tufa-forming organisms of tubular
shape were observed on the PL which was placed in
complete darkness. Furthermore, tufa grew faster on
the lateral walls which are more exposed to the light
and rich with moss than in the locations within
complete darkness and on smooth surfaces of PLs.
The subject of the next paper will be the
comparison of derived 3D models at all levels of
research and the calculation of the volumetric (mm
3
a
-1
) and linear (mm a
−1
) tufa growth rates. Also, in
future research, we are considering implementing a
hyperspectral camera at the micro and meso level of
research in order to analyze spectral reflection and
detect the plant fragments and macroinvertebrates
accumulated on the tufa surface.
ACKNOWLEDGEMENTS
This research was performed within the project UIP-
2017-05-2694 financially supported by the Croatian
Science Foundation. Authors would like to thank
administration of the NP Krka.
REFERENCES
Arenas, C., Vázquez‐Urbez, M., Auqué, L., Sancho, C.,
Osácar, C.,Pardo, G. (2014): Intrinsic and extrinsic
controls of spatial and temporal variations in modern
fluvial tufa sedimentation: A thirteen - year record from
a semi-arid environment, Sedimentology, 61(1), 90-132.
Arenas, C., Osácar, C., Sancho, C., Vázquez-Urbez, M.,
Auqué, L., Pardo, G. (2010). Seasonal record from
recent fluvial tufa deposits (Monasterio de Piedra, NE
Spain): sedimentological and stable isotope data,
Geological Society, London, Special Publications,
336(1), 119-142.
Auqué, L., Arenas, C., Osácar, C., Pardo, G., Sancho, C.,
Vázquez-Urbez, M. (2014). Current tufa sedimentation
in a changing-slope valley: the River Añamaza (Iberian
Range, NE Spain), Sedimentary Geology, 303, 26-48.
Barešić, J., Faivre, S., Sironić, A., Borković, D., Lovrenčić
Mikelić, I., Drysdale, R.N., Krajcar Bronić, I. (2021):
The potential of tufa as a tool for paleoenvironmental
research-a study of tufa from the Zrmanja river canyon,
Croatia, Geoscience, 11, 376.
Capezzuoli, E., Gandin, A., Pedley, M. (2014): Decoding
tufa and travertine (fresh water carbonates) in the
sedimentary record: the state of the art, Sedimentology,
61(1), 1-21.
Cukrov, N., Surić, M., Fuček, L., Ćosović, V., Korbar, T.,
Juračić, M. (2010). Geology of Krka River estuary, u:
Vodič ekskurzija–Excursion Guide-book (4. hrvatski
geološki kongres), Hrvatski geološki institut.
Bonacci, O., Roje-Bonacci, R., Andrić, I. (2017). Prilog
izučavanju hidrologije Skradinskog buka na rijeci Krki,
Hrvatske vode, 25(99), 27-36.
Domazetović, F., Šiljeg, A., Marić, I. (2020) Guidelines for
optimization of terrestrial laser scanning surveys over
gully erosion affected areas. In: Massimiliano Alvioli,
Ivan Marchesini, Laura Melelli & Peter Guth, eds.,
Proceedings of the Geomorphometry 2020 Conference,
220-223.
Drysdale, R., Gillieson, D. (1997). Micro‐erosion meter
measurements of travertine deposition rates: a case
study from Louie Creek, Northwest Queensland,
Australia. Earth Surface Processes and Landforms: The
Journal of the British Geomorphological Group,
22(11), 1037-1051.
Ford, T. D., Pedley, H. M. (1996). A review of tufa and
travertine deposits of the world, Earth-Science
Reviews, 41(3-4), 117-175.
Holjevac, N., Kuzle, I. (2019). Prvi cjeloviti višefazni
elektroenergetski sustav na svijetu–Krka Šibenik.
Annual of the Croatian Academy of Engineering,
2019(1), 162-174.
James, M. R., Chandler, J. H., Eltner, A., Fraser, C., Miller,
P. E., Mills, J. P., Noble, T., Robson, S., Lane, S. N.
(2019). Guidelines on the use of structure from motion
Multi-Resolution Modeling of the Tufa Formation Dynamic using Close-Range Photogrammetry, Handheld 3D Scanner and Terrestrial
Laser Scanner
81