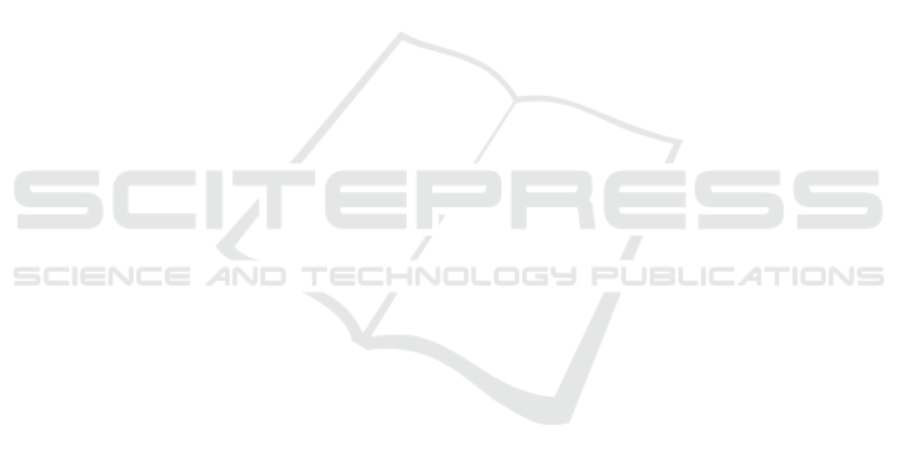
Landaluce, H., Arjona, L., Perallos, A., Falcone, F., Angulo,
I., and Muralter, F. (2020). A review of iot sensing
applications and challenges using RFID and wireless
sensor networks. Sensors (Switzerland), 20(9):1–18.
Magiera, A. and Solecka, J. (2020). Radiofrequency elec-
tromagnetic radiation from Wi-fi and its effects on
human health, in particular children and adolescents.
Review. Roczniki Panstwowego Zakladu Higieny,
71(3):251–259.
Maksimovi
´
c, M. and Vujovi
´
c, V. (2017). Internet of Things
Based E-health Systems: Ideas, Expectations and
Concerns.
Memon, S. F., Memon, M., and Bhatti, S. (2020). Wear-
able technology for infant health monitoring: A sur-
vey. IET Circuits, Devices and Systems, 14(2):115–
129.
Movassaghi, S., Abolhasan, M., Lipman, J., Smith, D., and
Jamalipour, A. (2014). Wireless Body Area Networks:
A Survey. IEEE Communications Surveys & Tutori-
als, 16(3):1658–1686.
Niemel
¨
a, V., Haapola, J., H
¨
am
¨
al
¨
ainen, M., and Iinatti, J.
(2017). An Ultra Wideband Survey: Global Regu-
lations and Impulse Radio Research Based on Stan-
dards. IEEE Communications Surveys and Tutorials,
19(2):874–890.
Pardal, M. L. and Marques, J. A. (2010). Towards the in-
ternet of things: An introduction to RFID technol-
ogy. Proceedings of the 4th International Workshop
on RFID Technology - Concepts, Applications, Chal-
lenges, IWRT 2010, in Conjunction with ICEIS 2010,
pages 69–78.
Philip, N. Y., Rodrigues, J. J., Wang, H., Fong, S. J., and
Chen, J. (2021). Internet of Things for In-Home
Health Monitoring Systems: Current Advances, Chal-
lenges and Future Directions. IEEE Journal on Se-
lected Areas in Communications, 39(2):300–310.
Qu, Q., Li, B., Yang, M., Yan, Z., Yang, A., Yu, J., Gan, M.,
Li, Y., Yang, X., Aboul-Magd, O., Au, E., Deng, D. J.,
and Chen, K. C. (2018). Survey and performance eval-
uation of the upcoming next generation WLAN stan-
dard - IEEE 802.11ax. arXiv, 1:1461–1474.
Roy, S. and Chowdhury, C. (2021). Remote health mon-
itoring protocols for IoT-enabled healthcare infras-
tructure. In Healthcare Paradigms in the Internet of
Things Ecosystem, pages 163–188. Elsevier.
Seferagi
´
c, A., Famaey, J., De Poorter, E., and Hoebeke,
J. (2020). Survey on wireless technology trade-offs
for the industrial internet of things. Sensors (Switzer-
land), 20(2):1–22.
Thomas, D., Wilkie, E., and Irvine, J. (2016). Compar-
ison of Power Consumption of WiFi Inbuilt Inter-
net of Things Device with Bluetooth Low Energy.
10(10):1693–1696.
Ullah, S., Mohaisen, M., and Alnuem, M. A. (2013). A re-
view of IEEE 802.15.6 MAC, PHY, and security spec-
ifications.
Vidakis, K., Mavrogiorgou, A., Kiourtis, A., and Kyri-
azis, D. (2020). A Comparative Study of Short-Range
Wireless Communication Technologies for Health In-
formation Exchange. 2nd International Conference on
Electrical, Communication and Computer Engineer-
ing, ICECCE 2020, (June).
Vyas, A., Pal, S., and Saha, B. K. (2021). Relay-based
Communications in WBANs. ACM Computing Sur-
veys, 54(1):1–34.
Watson, A. R., Wah, R., and Thamman, R. (2020). The
value of remote monitoring for the COVID-19 pan-
demic. Telemedicine and e-Health, 26(9):1110–1112.
WiMedia Alliance (2009). Multiband Ofdm Physical Layer
Specification. (1.5):204.
Yu-nan, H., Ying-hua, L., Jin-ling, Z., and Xiao-lu, Z.
(2006). The Interaction between PIFA for Bluetooth
applications and a High-Fidelity Human Body Model.
In The 2006 4th Asia-Pacific Conference on Environ-
mental Electromagnetics, pages 331–335. IEEE.
Ziegelberger, G., Croft, R., Feychting, M., Green, A. C.,
Hirata, A., D’Inzeo, G., Jokela, K., Loughran, S.,
Marino, C., Miller, S., Oftedal, G., Okuno, T., van
Rongen, E., R
¨
o
¨
osli, M., Sienkiewicz, Z., Tattersall,
J., and Watanabe, S. (2020). Guidelines for Limiting
Exposure to Electromagnetic Fields (100 kHz to 300
GHz). Health Physics, 118(5):483–524.
Zigbee Alliance (2008). Zigbee Specification. Zigbee Al-
liance website, pages 1–604.
Zradzi
´
nski, P., Karpowicz, J., Gryz, K., Morzy
´
nski, L.,
Mły
´
nski, R., Swidzi
´
nski, A., Godziszewski, K., and
Ramos, V. (2020). Modelling the influence of elec-
tromagnetic field on the user of a wearable iot de-
vice used in a WSN for monitoring and reducing haz-
ards in the work environment. Sensors (Switzerland),
20(24):1–15.
WINSYS 2021 - 18th International Conference on Wireless Networks and Mobile Systems
106