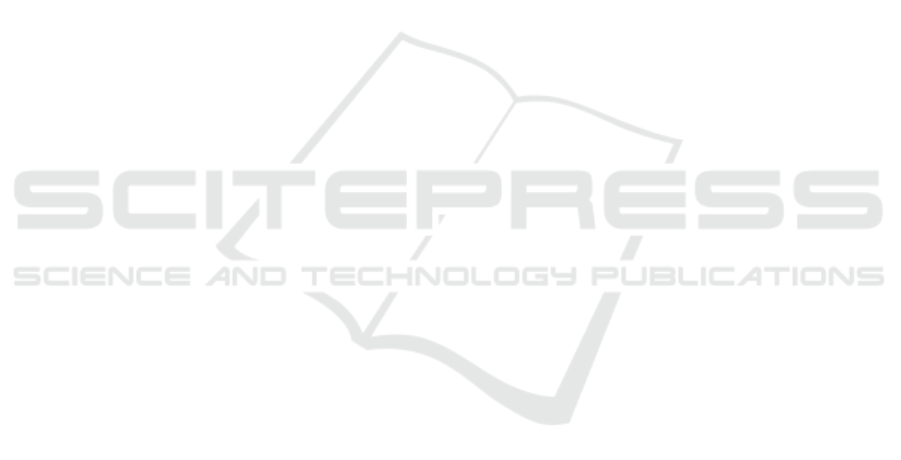
using hygienic and clean textile materials which is
increasing. Textile materials, especially for
underwear, are indeed susceptible to exposure, and
can even be a living medium for microbes, which
cause these textile materials: easy to smell, have
spots, and damage (Kumar, 2011).
Cellulose is one of the most widely dispersed,
renewable, and biodegradable polymers. Cellulose is
a natural polymer that does not dissolve in water due
to long chains and high molecular weight (more than
500,000 Da) (Fathanah, Lubis, Othman, Handayani,
& Karlina, 2017).
Cellulose is the main element of all plant material,
forming about half to one third of all plant tissue and
is constantly formed from photosynthesis (Sun, Sun,
Zhao, & Sun, 2004). This is the main structural
component that provides strength and stability to plant
cell walls which are arranged in microfibrils in cell
walls, interrupted by hemicellulose and surrounded by
a lignin matrix. Depending on the type of plant, most
plant material consists of about 40 - 55% cellulose, 15
- 35% lignin and 25 - 40% hemicellulose. On the cell
wall of natural plants, cellulose crystals are covered
with these substances which makes it difficult to get
pure cellulose. Chemically, cellulose is a naturally
occurring linear polymer of anhydroglucose unit
connected to one and four carbon atoms by β-
glycosidic bonds. It is proven by the presence of three
hydroxyl (OH) groups with different acidity /
reactivity, where secondary OH is located in the C-2,
and C-3 positions, and the primary OH is located in
the C-6 position. This is also verified by the formation
of various strong hydrogen bonds between molecules
and intramolecules (Penjumras, Abdul, Talib, &
Abdan, 2014). In recent years, interest in cellulose-
based materials has increased due to the demand for
renewable resources (Mohanty et al., 2005).
MA grafting into various polymers has been
popular for the past 20 years. Grafted copolymers
can be used as the main component. Various
polymers have been used as backbones for grafting
maleic anhydride. Other types of thermoplastics (PS,
EVA, PES), as well as elastomers (EPDM, EPR,
NR), can also be used as polymers for MA grafting
(Krump, Alexy, & Luyt, 2005).
As is known, 2-acryloyloxyethyl trimethyl
ammonium chloride (AETAC) consists of
quaternary ammonium salt groups and unsaturated
vinyl groups. Vinyl groups can be polymerized and
can react with a variety of vinyl monomers, which in
2016 Shen et al conducted research on poly [(2-
acryloyloxyethyl trimethyl ammonium chloride) -co-
(acrylic acid)] branches onto starch for cotton warp
sizing (Shen, Zhu, & Liu, 2016).
Wool fiber antimicrobial textile material has
been developed using a coating technique with a
bioactive polymer material, [2- (acryloiloxy) ethyl]
trimethylammonium chloride (AETAC).
Antimicrobial properties of wool fiber coated with
bioactive polymers were tested against Gram-
positive and Gram-negative bacteria,
Staphylococcus aureus and Klebsiella pneumoniae.
Furthermore, the surface morphology characteristics
of the fibers were tested using reflected infrared
spectroscopy (ATR-FTIR), electron microscopy
(SEM), tensile strength and contact angle to water
drops. the result is that Q-chitosan-based
antibacterial properties against wool can be
improved by grafting wool fabric with polystyrene
sulphonate (pSS), which shows very good
antibacterial activity against Staphylococcus aureus
and Klebsiella but less good against the fungus
Aspergillus fumigatus. Transplanting a wool fabric
with pSS not only increases the tensile strength of
the fabric but also increases the durability of the
treatment of several washings. pSS-g-wool, Q-
chitosan evenly covers the surface of the treated
fiber and after 5x the washing is still intact.
Therefore this grafting process is commercially
viable for wool fabrics (Hassan, 2015).
The two most common methods used to analyze
thermal properties are thermogravimetric analysis
(TGA) and (DSC). The TGA technique measures the
weight loss and mass change of a substance as a
function of temperature. However, there are several
reactions that can occur without mass loss. In this
case, DSC is able to detect these reactions
(Zakikhani, 2016).
In this research, the surface modification of
cellulose fiber from Belangke bamboo will be
modified with antimicrobial compound (AETAC),
with the help of maleic anhydride (AM) and
ammonium persulfate as comonomer and initiator.
Furthermore, the surface morphology characteristics
of the fibers were tested using reflected infrared
spectroscopy (ATR-FTIR), electron microscopy
(SEM), Thermal Analysis Differential Scanning
Calorimetry (DSC).
2 MATERIALS AND METHODS
2.1 Materials
The material used in this study is 80% solution of 2-
(acryloyloxy)-ethyltrimethylammonium chloride were
purchased from Sigma-Aldrich Chemicals (USA) and
used without any purification, ammonium persulfate
ICOCSTI 2019 - International Conference on Chemical Science and Technology Innovation
306