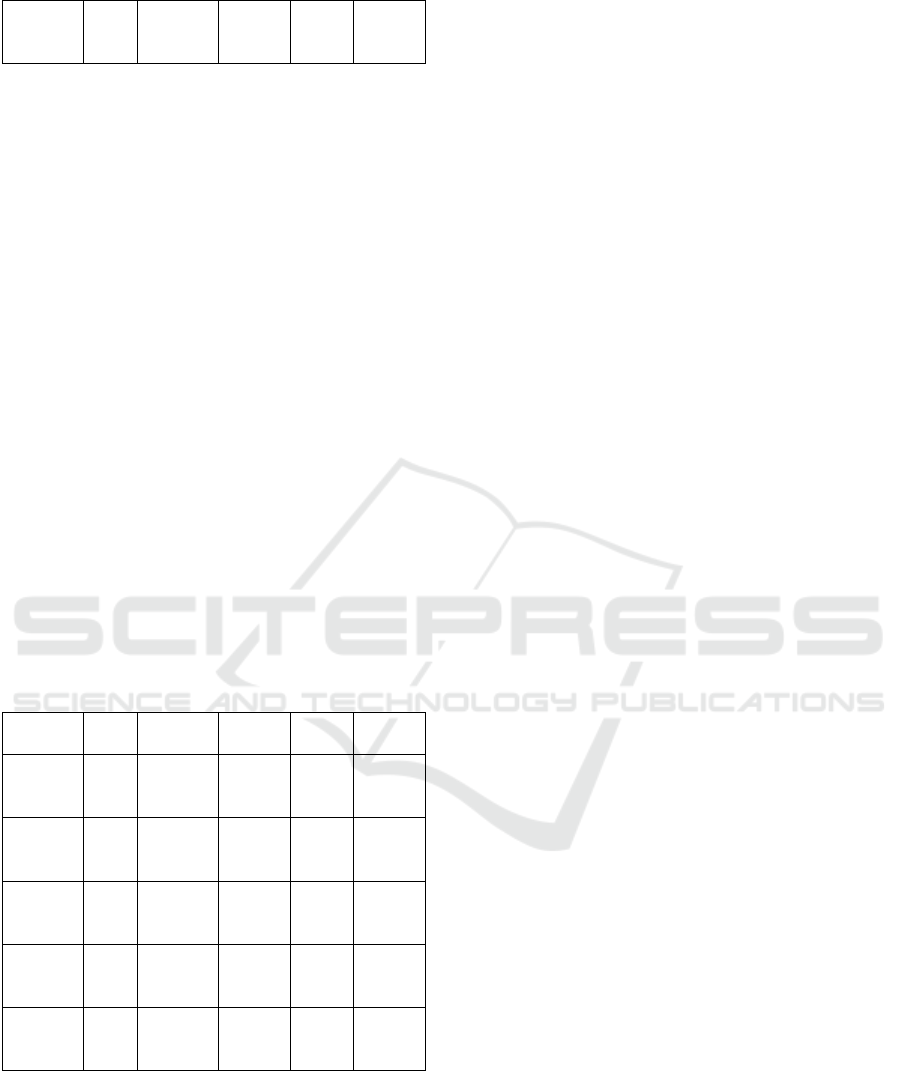
The adsorption capacity of pillared bentonite
shows greater value at high concentration of Congo
red for all temperature measured. Pillarization
treatment was able to increase basal spacing between
layers of bentonite hence the capacity of pillared
bentonite to adsorb is bigger.
Result of energy calculation exposes that the
adsorption energy is positive and increase as
temperature rise. Normally, adsorption is an
exothermic event hence has negative energy. The
unbalance energy at surface was compensated by
adsorbing solid adsorbate so it decreases its energy.
The situation for adsorption in solution however
must take into account solvent contribution.
Fontecha-Ca´mara et al. (2006) suspected that the
interaction of solvent-adsorbent and solvent-
adsorbate could shifts the exothermic mode of
adsorption into endothermic one.
The calculated parameters of thermodynamic
based on adsorption of direct violet at various
temperatures is displayed on Table 7. The adsorption
was conducted over Fe
3+
/Ti
4+
pillared bentonite.
Table 7: Adsorption energy (E), entropy (∆S), enthalpy
(∆H) and adsorption capacity (qe) of direct
violet adsorption over Fe3+/Ti4+ pillared
bentonite at various temperature
The Gibbs free energy (∆G) for both Congo red and
direct violet according to calculation result is
negative which means it occurs spontaneously.
Table 7 shows similar trends with previous table.
Entropy value obtained nonetheless shows large
difference. As temperature increase, so does entropy
of both adsorptions. Direct violet appears slightly
smaller entropy compare to Congo red. The
complexity of adsorbate structure might hinder the
adsorption process due to steric hindrance so it
lowers entropy of the process. Enthalpy result is
quite similar as Congo red. The type of interaction
for adsorption in solution possess was difficulty and
not as simple as gas adsorption over solid adsorbent.
4 CONCLUSIONS
Basal spacing of natural bentonite was successfully
increased from 13.8 to 15 Å through pillarization
using Fe
3+
/Ti
4+
at 2:1 ratio. Quantitatively,
spectrophotometer UV-Vis confirmed the dyes
removal from decrease of intensity but FTIR spectra
gave no peaks indication of the functional groups of
corresponding dyes. The optimum adsorption
condition is in accordance with pH
pzc
i.e. higher at
pH below pH
pzc
. Freundlich model for heterogenic
surface along with possible chemical interaction is
best fit to the process conducted. Thermodynamic of
adsorption suggest the process is endothermic and
occurred spontaneously.
ACKNOWLEDGEMENTS
The first author want to say thank you to
KEMENRISTEKDIKTI for the financial support
through Hibah Penelitian Dasar 2019.
REFERENCES
Bentahar, S., Abdellah, D., Mohammed, E.L., and
Noureddine, E.M., 2017. Adsorption of Methylene
Blue, Crystal Violet and Congo red From Binary and
Ternary Systems with Natural Clay: Kinetic, Isotherm,
and Thermodynamic. Journal of Environmental
Chemical Engineering. 5: 5921-5932.
Chinoune, K., Bentaleb, K., Bouberka, Z., Nadim, A., and
Maschke, U., 2016. Adsorpsi of Reactive Dyes from
Aqueous Solution by Dirty Bentonite. Applied Clay
Science. 132: 64-75.
Cool, P., and Vansant, E.F., 1998. Pillared Clays:
Preparation, Characterization and Applications.
University of Antwerp (UIA): Laboratory of Inorganic
Chemistry, Department of Chemistry.
Fabryanty, R., Valencia, C., Soetaredjo, F.E., Putro, J.N.,
Santoso, S.P., Kurniawan, A., Ju, Y.H., and Ismadji,
S., 2017. Removal of Crystal Violet Dye by
Adsorption Using Bentonite – Alginate Composite.
Pillarization of Bentonite using Fe3+/Ti4+ and Its Application for Congo Red and Direct Violet Removal
87