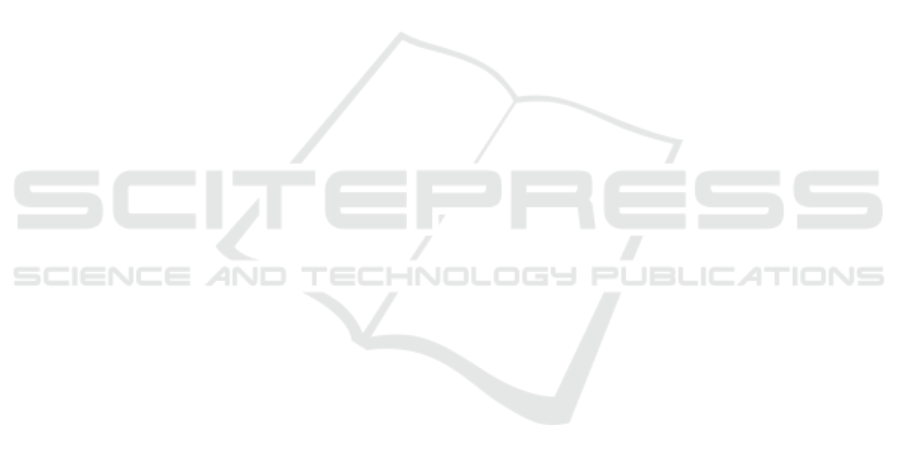
2 MATERIAL AND METHOD
2.1 Materials
Three salinity genes on oil palm acquired from NCBI.
The DNA and amino acid references used in the
research follow:
1. XM_019850660.1 GI: 1130661850 Salt stress-
induced hydrophobic peptide ESI3-like [E.
guineensis]; NCBI Reference Sequence:
XP_019706219.1
2. XM_019846899.1 GI: 1130626415 Salt stress-
induced hydrophobic peptide ESI3-like [E.
guineensis]; NCBI Reference Sequence:
XP_019702458.1
3. JZ142439.1 GI: 527498159 EgFLSTP6 E.
guineensis cDNA similar to salt tolerance protein
6, mRNA sequence 525 bp linear mRNA
2.2 Physicochemical Features of the
Salinity Protein of Oil Palm
Online Protparam (web.expasy.org/protparam/) has
been used to control the composition,
physicochemical features of oil palm plants ' protein
salinity. The calculated factors are the length of
genes/bp, molecular weight, theoretical isoelectric
values points, a total number of atoms, total
negatively charged residues, total number of
positively charged residues, instability coefficient,
aliphatic index, and grand mean of hydropathicity as
prior described (Basyuni et al., 2017)
2.3 Peptide Transfer and Subcellular
Localization of Protein-induced
Salinity Proteins in Oil Palm Plants
Peptide predictions transit through the online P1.1
target server (www.cbs.dtu.dk/services/targetp/).
Peptide chloroplast transit, mitochondrial target
peptide, the signal peptide of the secretory pathway,
reliability indicator were found. Online PSORT
(predictive tool for subcellular localization of
proteins) (psort.hgc.jp/form.html) is used to analyze
the subcellular of protein dehydration which is
induced by salinity stress in oil palm plants as earlier
shown (Basyuni and Wati, 2017).
2.4 Phylogenetic Analysis of Protein-
induced Salinity in Oil Palm
Locus numbers of the sequence of the physical and
chemical characteristic of oil palm protein induced
salinity used this investigation in this manner:
E.guineensis XM_019850660, XM_019846899, and
JZ142439.1. Phylogenetic analysis of amino acid
arrangement from of protein-induced salinity in oil
palm was carried out with CLUSTAL W ver. 1.83
(Thompson et al., 1994) of the DNA Data Bank of
Japan (Mishima, Shizuoka, Japan) accompanied by
depicting with TreeView, ver. 1.6.6 (Page, 1996)
according to a neighbor-joining method. Bootstrap
analysis with 1000 repetitions was used to weigh the
strong point of the knots in the tree (Felsenstein.
1995).
3 RESULT
3.1. Physicochemical Characteristics of
the Protein Induced Salinity in E.
guineensis
The physicochemical activities of a protein are
analyzed by the similar properties of the amino acids
in it. Each protein molecule contains of a long chain
of amino acid residues and is connected by peptide
bonds. Table 1 showed the several parameters of
physicochemical protein induced salinity in
E.guineensis. Length of genes between 525 to 633 bp.
The same Molecular weight at X1 and X2 is 5945.24,
but it is different from X3 which is 18052.17.
Theoretical isoelectric points values at X1 and X2 are
almost close to 4.65 and 4.0 but different from X3
which is 8.69. The total number of atoms at X1 and
X2 is almost close to 8.64 and 8.71 three times
different from X3 which is 2478.
The total number of negatively charged residues
X1 and X2 which are 3 and 4 are different from X3
which is 11. The total number of positively charged
residues on X1 and X2 is the same, namely 1, but
different from X3, namely 14, Instability coefficient
at X1, X2 and X3 between 27.15 and 31.66. Aliphatic
indexes at X1 and X2 are 162.42 and 165.93 but are
low at X3 which is 60.18. The hydrophobicity is a
significant stabilization force in protein folding.
Grand average of hydropathicity on X1 and X2 is
1.604 and 1.491 while X3 is -0.443. Despite the
availability of thousands of stress associated ESTs of
in E. guineensis (Low et al., 2008), quantitative gene
expression analysis of these genes is only recently
attempted for the identification of candidate
genes/factors that are contributing to salinity
tolerance. With the advent of the qPCR technique, it
is easier to quantify each gene and establish its
relevance under the given stress situations.
Protein Induced as Salinity Stress in Elaeis guineensis Jacq.
113