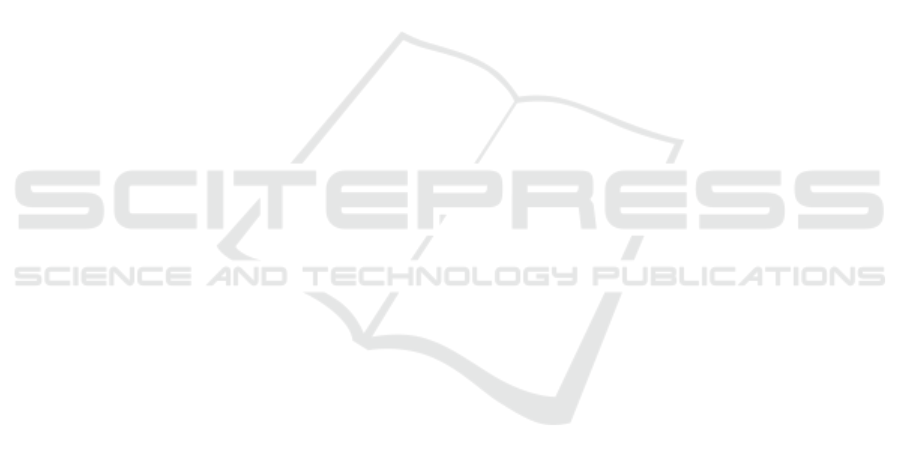
4 CONCLUSIONS
Our research point out that, increasing the intensity of
irradiation reducing the germination time from 197
days (without treatment) become 61 days (dose 40
Gy). Increasing irradiation intensity also decreasing
the sprouting ability from 83.8% (without treatment)
to 75.0% and 57.5% (dose 10 and 20 Gy). The
irradiation also affects the number of leaves. The
number of leaves was 10 sheets (without treatment)
but decreases after irradiated at an intensity of 10 Gy
to 8 sheets. The irradiation treatment also affects plant
height. But statistically the response of the two
treatments is the same. Conversely, irradiation
treatment does not affect stem diameter and number
of branches.
ACKNOWLEDGEMENTS
I would like to extend thanks to Wijaya Murti
Indriatama, Agriculture researcher on National
Atomic Energy Agency (PAIR BATAN).
REFERENCES
Akshatha, Chandrashekar, K. R., Somashekarappa, H. M.,
Souframanien, J. 2013. Effect of gamma irradiation on
germination, growth, and biochemical parameters of
Terminalia arjuna Roxb. Radiation Protection and
Environment, 36(1), 3844.
BPS Provinsi Sumatera Utara. 2013. North Sumatera in
Figure. Medan.
Busby, B. 2008. Radiation and radioactivity. Akses tanggal
2 November 2012, dari http://Physics. isu.edu/
radinf.htm.
Chan, Y. K., Lam, P. F. 2002. Irradiation-induced
mutations in papaya with special emphasis on papaya
ringspot resistance and delayed fruit ripening. Working
Material – Improvement of tropical and subtropical
fruit trees through induced mutations and
biotechnology. Vienna, Austria: IAEA, pp 35-45.
De Micco, V., Arena, D., Pignalosa, D., Durante, M. 2011.
Effects of sparsely and densely ionizing radiation on
plants. Radiat. Environ. Biophys., 50, 1-19.
Esnault, M. A., Legue, F., Chenal, C. 2010. Ionizing
radiation: Advances in plant response. Environ. Exp.
Bot., 68, 231-237.
Gruner, M. M., Horvatic, D., Kujundzic, Magdalenic, B.
1992. Effect of gamma irradiation on the lipid
components of soy protein products. Food and nutrition
bulletin supplement/Nahrung, 36: 443-450.
Habba, I. E. 1989. Physiological effect of gamma rays on
growth and productivity of Hyoscyamus muticus L. and
Atropa belladonna L. Ph.D. Thesis, Fac. Agric. Cairo
Univ., Cairo, Egypt. 65-73.
Hapsari, L. 2004. Mutation Induction on Melati (Jasminum
spp.) Using Gamma Ray Induction. Skripsi.
Departemen Budidaya Pertanian, Fakultas Pertanian,
Bogor Agricultural University. Bogor.
Hartati, S. 2000. Genotipic Perform of Tomat
(Lycopersicum esculentum Mill.) Mutation in Different
Water Stress Condition and Optimal Condition.
Agrosains 2 (2): 35-42.
Herison, C., Rustikawati, S. H. Sutjahjo, S. I. Aisyah. 2008.
Gamma ray mutation induction on Zea mays to
increasing its diversity Jurnal Akta Agrosia, 11(1):
5762.
Iglesias-Andreu, L. G., Octavio-Aguilar, P., BelloBello, J.
(2012). Current importance and potential use of low
doses of gamma radiation in forest species In Gamma
radiation. (Adrovic, F., Ed.). . Rijeka, In Tech Europe
Croatia. p. 265-280.
Luckey, T. 1998. Radiation hormesis: Biopositive effect of
radiation. Radiation Science and Health.Boca Raton,
FLO, USA: CRC press.
Luckey, T. 1998. Radiation hormesis: Biopositive effect of
radiation. Radiation Science and Health. CRC press.
Boca Raton, FLO, USA. Marcu, D., Cristea, V., and L.
Daraban. 2012. Dose-dependent effects of gamma
radiation on lettuce (Lactuca sativa var. capitata)
seedlings. International Journal of Radiation Biology,
1–5.
Luckey, T. 2003. Radiation hormesis overview. RSO
Magazine 4:19–36
Marcu, D., Cristea, V., Daraban, L. 2013. Dose-dependent
effects of gamma radiation on lettuce (Lactuca sativa
var. capitata) seedlings. International Journal of
Radiation Biology, 89(3), 219-223.
Piri, I., Babayan, M., Tavassoli, A. and Javaheri, M. 2011.
The use of gamma irradiation in agriculture. African
Journal of Microbiology Research 5(32):5806-5811.
Susilowati, A., Kholibrina C. R., Rachmat H. H., Munthe,
M. A. 2018. Phylogeny of Kemenyan (Styrax sp) Based
on Morphological Character. IOP Conf. Ser.: Earth
Environ. Sci. 122 012062.
World Health Organization (WHO). 1988. Food irradiation:
A technique for preserving and improving the safety of
food. WHO Publication in Collaboration with FAO. pp.
144-149.
Wulandari, A. 2001. Induction and mutation of Krisan
(Dendrathema grandiflora) using irradiation shoot
cutting. Skripsi. Departemen Budidaya Pertanian,
Fakultas Pertanian, Institut Pertanian Bogor. Bogor.
Hal 19-20.
Zanzibar, M., Sudrajat, D.J. 2015. Effect of Gamma
Irradiation on Seed Germination, Storage, and Seedling
Growth of Magnolia champaca (L.) Baill. ex Pierre.
Zanzibar, M., Witjaksono. 2011. The effect of aging and
seed irradiation using gamma ray (60C) on suren
(Toona sureni Blume Merr) growth. Jurnal Penelitian
Hutan Tanaman, 8.2: 89-96.
Gamma Ray Application for Increasing Kemenyan Toba (Styrax sumatrana) Seed Viability
23