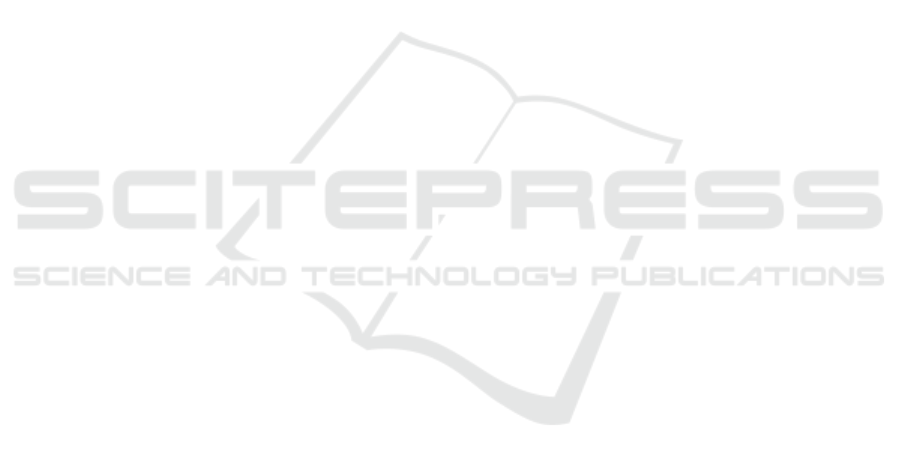
judgements, future research should integrate
individual factors to develop more target-group
oriented recommendations and communication
strategies (e.g., Arning et al., 2018). Further, the study
should be replicated with a larger representative
sample in Germany, but also with international
samples to allow cross-cultural comparisons of AF
production plant infrastructure acceptance.
5 CONCLUSIONS
The present study successfully identified and
assessed acceptance-relevant factors with regard to
AF production plant infrastructure design. Although
the integration of acceptance evaluations as soft
factor into power design planning tools needs further
methodological refinement, insights on drivers of AF
production plant infrastructure acceptance were
gained, which allowed to simulate preferences for
future AF production plant roll-out scenarios.
ACKNOWLEDGEMENTS
This work was supported by the Cluster of Excellence
“Tailor-Made Fuels from Biomass”, which is funded
under Contract EXC 236 by the Excellence Initiative
by the German federal and state governments to
promote science and research at German universities.
REFERENCES
Arning, K. (2017). Conjoint Measurement. The
International Encyclopedia of Communication
Research Methods, 1-10. Wiley.
Arning, K., van Heek, J., & Ziefle, M. (2018). Acceptance
profiles for a carbon-derived foam mattress. Exploring
and segmenting consumer perceptions of a carbon
capture and utilization product. Journal of Cleaner
Production, 188, 171-184.
Batel, S., Devine-Wright, P., & Tangeland, T. (2013). Social
acceptance of low carbon energy and associated infra-
structures: A critical discussion. Energy Policy, 58, 1-5.
Borning, M., van Bracht, N., Moser, A., Doré, L., Wolff,
M., & Walther, G. (2018). Electricity-Based Fuel
Production: An Assessment of its Impact on the
European Power System Considering Flexibility. In
2018 15th International Conference on the European
Energy Market, IEEE.
Cetinay, H., Kuipers, F. A., Guven, A. N. (2017). Optimal
siting and sizing of wind farms. Renewable Energy,
101, 51-58.
Chin, H. C., Choong, W. W., Alwi, S. R. W., &
Mohammed, A. H. (2014). Issues of social acceptance
on biofuel development. Journal of cleaner production,
71, 30-39.
Chu, S., Majumdar, A. (2012). Opportunities and
challenges for a sustainable energy future. Nature, 488,
294–303.
dena (2017). E-Fuels – The potential of electricity-based
fuels for low emission transport in the EU. Deutsche
Energie-Agentur GmbH (dena). Berlin.
Fortenbery, T. R., Deller, S. C., & Amiel, L. (2013). The
location decisions of biodiesel refineries. Land
Economics, 89(1), 118-136.
Hackbarth, A., & Madlener, R. (2016). Willingness-to-pay
for alternative fuel vehicle characteristics: A stated
choice study for Germany. Transportation Research
Part A: Policy and Practice, 85, 89-111.
Lee, G. E., Loveridge, S., & Joshi, S. (2017). Local
acceptance and heterogeneous externalities of
biorefineries. Energy Economics, 67, 328-336.
Luenberger, D. G., Ye, Yinyu (2016). Linear and Nonlinear
Programming. Springer International Publishing.
Moula, M. M. E., Nyári, J., & Bartel, A. (2017). Public
acceptance of biofuels in the transport sector in Finland.
International Journal of Sustainable Built Environment,
6(2), 434-441.
Plec, E., & Pettenger, M. (2012). Greenwashing
consumption: The didactic framing of ExxonMobil's
energy solutions. Environmental Communication: A
Journal of Nature and Culture, 6(4), 459-476.
Rao, V. R. (2014). Applied conjoint analysis. New York,
NY: Springer.
Roche, M. Y., Mourato, S., Fischedick, M., Pietzner, K., &
Viebahn, P. (2010). Public attitudes towards and
demand for hydrogen and fuel cell vehicles: A review
of the evidence and methodological implications.
Energy Policy, 38(10), 5301-5310.
Santibañez-Aguilar, J. E., González-Campos, J. B., Ponce-
Ortega, J. M., Serna-González, M., & El-Halwagi, M.
M. (2014). Optimal planning and site selection for
distributed multiproduct biorefineries involving
economic, environmental and social objectives. Journal
of cleaner production, 65, 270-294.
Sawtooth, 2009. Introduction to Market Simulators for
Conjoint Analysis. [WWW Document]. URL
https://www.sawtoothsoftware.com/download/techpap
/introsim.pdf (accessed 2.22.19).
Sawtooth, 2017. The CBC System for Choice-Based
Conjoint Analysis. Technical Paper Version 9 [WWW
Document]. URL https://sawtoothsoftware.com/down
load/techpap/cbctech.pdf (accessed 2.22.19).
Skar, C., Doorman, G. L., Tomasgard, A. (2014). The
future European power system under a climate policy
regime. ENERGYCON 2014: IEEE International
Energy Conference.
Soland, M., Steimer, N., & Walter, G. (2013). Local
acceptance of existing biogas plants in Switzerland.
Energy Policy, 61, 802-810.
Stephanopoulos, G. (2007). Challenges in engineering
microbes for biofuels production. Science, 315(5813),
801-804.
SMARTGREENS 2019 - 8th International Conference on Smart Cities and Green ICT Systems
98