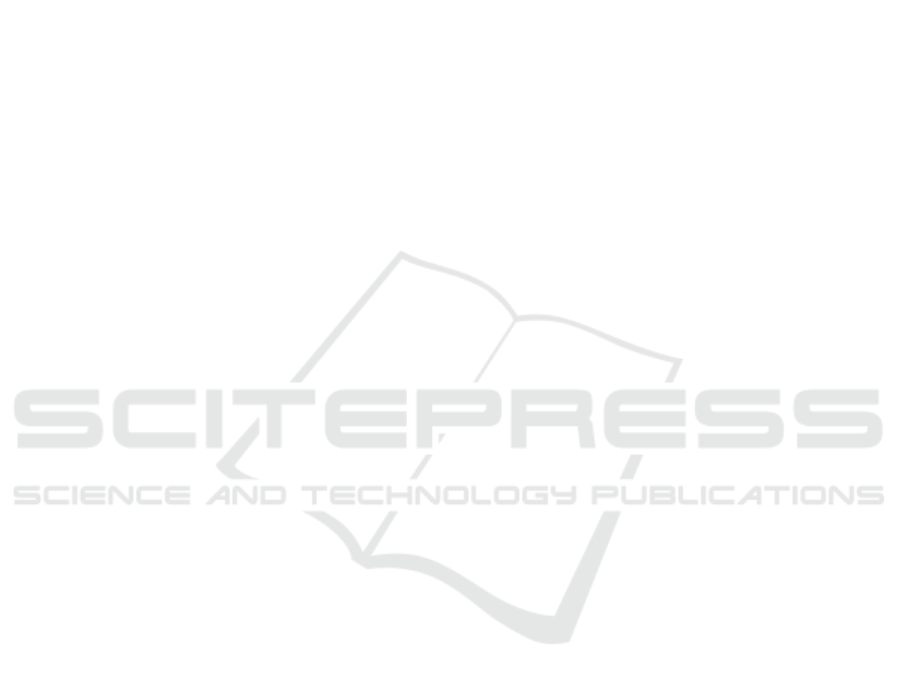
ACKNOWLEDGEMENTS
This work was supported in part by the Ministry of
Science and Technology, TAIWAN, numbers MOST
106-2221-E-492 -012 and 107-2622-E-492 -009 -
CC3
REFERENCES
COHERENT, Co. Ltd., 2015. White paper: The digital
display revolution: Built on Excimer laser annealing.
Zhang, K., Li, S., Liang, G., Huang, H., Wang, Y., Lai, T.,
Wu, Y., 2012. Different crystallization processes of as-
deposited amorphous Ge
2
Sb
2
Te
5
films on nano-and
picoseconds single laser pulse irradiation. Physica B,
Vol. 407, pp. 2447-2450.
Huang, L., Jina, J., Shia, W., Yuanb, Z., Yanga, W.,
Caoa, Z., Wanga, L., Zhoub, J., Loub, Q., 2013.
Characterization and simulation analysis of laser-
induced crystallization of amorphous silicon thin films.
Mater. Sci. Semicond. Process. Vol. 16, pp. 1982-1987.
Kuo, C.C., Yeh, W.C., Lee, J.F., Jeng, J.Y., 2007. Effects
of Si film thickness and substrate temperature on melt
duration observed in excimer laser-induced
crystallization of amorphous Si thin films using in-situ
transient reflectivity measurements. Thin Solid Films
Vol. 515, pp. 8094-8100.
Emelyanov, A.V., Khenkin, M.V., Kazanskii, A.G., Forsh,
P.A., Kashkarov, P.K., Gecevicius, M., Beresna, M.,
Kazansky, P.G., 2014. Femtosecond laser induced
crystallization of hydrogenated amorphous silicon for
photovoltaic applications. Thin Solid Films Vol. 556,
pp. 410-413.
Cheng, C.W., Lee, I.M., Chen, J.S., 2014. Femtosecond
laser-induced nanoperiodic structures and simultaneous
crystallization in amorphous indium-tin-oxide thin
films. Appl. Surf. Sci. Vol. 316, pp. 9-14.
Lee, S., Seong, J., Kim, D.Y., 2010. Effects of laser-
annealing using a KrF excimer laser on the surface,
structural, optical, and electrical properties of AlZnO
thin films. J. Korean Phys. Soc. Vol. 56, pp. 782-786.
Tsang, W.M., Wong, F.L., Fung, M.K., Chang, J.C., Lee,
C.S., Lee, S.T., 2008. Transparent conducting
aluminum-doped zinc oxide thin films prepared by sol-
gel process followed by laser irradiation treatment. Thin
Solid Films Vol. 517, pp. 891-895.
Chen, M.F., Lin, K.M., Ho, Y.S., 2012. Laser annealing
process of ITO thin films using beam shaping
technology. Opt. Lasers Eng. Vol. 50 pp. 491-495.
Chen, M.F., Lin, K.M., Ho, Y.S., 2011. Effects of laser-
induced recovery process on conductive property of
SnO
2
:F thin films. Mater. Sci. Eng. B Vol. 176, pp. 127-
131.
Kim, M.G., Kanatzidis, M.G., Facchetti, A., Marks, T.J.,
2011. Low-temperature fabrication of high-
performance metal oxide thin-film electronics via
combustion processing. Nat. Mater. Vol. 10, pp. 382-
388.
Morimoto, K., Suzuki, N., Liu, X., Samonji, K., Yamanaka,
K., Yuri, M., 2012. Amorphous Si crystallization by
405 nm GaN laser diodes for high performance TFT
applications: advantages of using 405 nm wavelength.
Proc. of SPIE Vol. 8244, pp. 824407-1.
Jun, M.C., Park, S.U., Koh, J.H., 2012. Comparative
analysis of Al-doped ZnO and Ga-doped ZnO thin
films. Integr. Ferroelectr., Vol. 140, pp. 166-176.
Cebulla, R., Wendt, R., Ellmer, K., 1998. Al-doped zinc
oxide films deposited by simultaneous rf and dc
excitation of a magnetron plasma: relationships
between plasma parameters and structural and electrical
film properties. J. Appl. Phys. Vol. 83, pp. 1087-1095.
Hsiao, W.T., Tseng, S.F., Huang, K.C., Chiang, D., 2013.
Electrode patterning and annealing processes of
aluminum-doped zinc oxide thin films using a UV laser
system. Opt. Laser Eng. Vol. 51, pp. 15-22.
Huang, L.J., Li, B.J., Ren, N.F., 2016. Enhancing optical
and electrical properties of Al-doped ZnO coated
polyethylene terephthalate substrates by laser annealing
using overlap rate controlling strategy. Ceramic
International Vol. 42, pp. 7246-7252.
Jo, G.H., Kim, S.H., Koh, J.H., 2018. Enhanced electrical
and optical properties based on stress reduced graded
structure of Al-doped ZnO thin films. Ceramic
International Vol. 44, 735-741.
Xu, K., Huang, L., Zhang, Z., Zhao, J., Zhang, Z., Snyman,
L.W., Swart, J.W., 2018. Light emission from a poly-
silicon device with carrier injection engineering.
Materials Science & Engineering B Vol. 231, pp. 28-
31.
Kim, J., Ji, J.H., Min, S.W., Jo, G.H., Jung, M.W., Park,
M.J., Lee, S.K., Koh, J.H., 2017. Enhanced
conductance properties of UV laser / RTA annealed Al-
doped ZnO thin films. Ceramic International Vol. 43,
3900-3904.
PHOTOPTICS 2019 - 7th International Conference on Photonics, Optics and Laser Technology
138