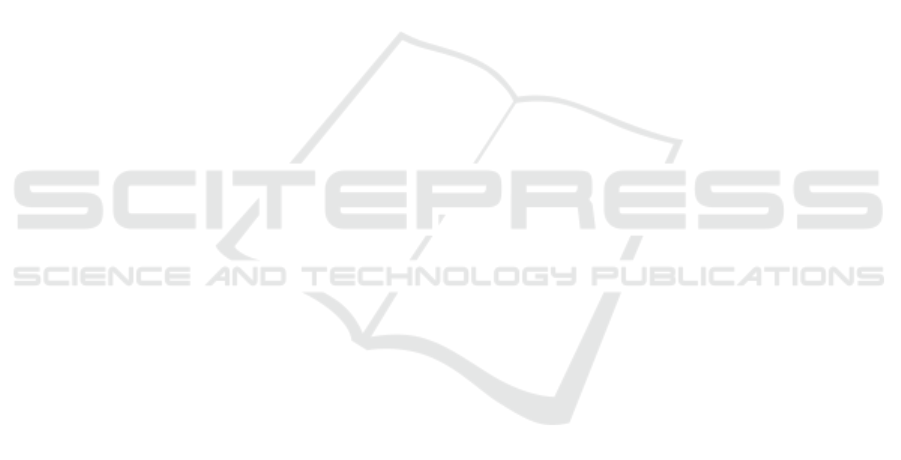
2.4 Energy Efficiency and CO
2
Emission Decrease
According to the majority of experts, the value of
"energy payback period" (EPBT) for an SPVP, in the
current phase of technology development, is about 1.3
years with a service life of at least 25 years
(Greenpeace, 2012). In fact, this is an equivalent of
Energy Return on investment (EROI) at 1:19, which
makes this technology equal in efficiency to oil and
gas upstream (from 1:19 to 1:32 depending on the
degree of recovery). Due to technological
improvement, this indicator for an SPVP has
significantly improved over the past 5 years.
According to some studies, any technology in the
energy sector must beat the 1:14 indicator to be
efficient in the long run (Lambert et al., 2012). Thus,
it can be stated that, during approximately 23 of 25
years of an SPVP’s lifecycle, it generates electricity
without any emissions into the environment.
Several sources say that the harmful emissions of
SPVPs, based on the most energy-consuming
technology of crystalline silicon, are equal to 33-50
g/kWh of CO
2
equivalent (for thin-film technologies,
this value is 18 g/kWh), while coal-consuming power
plants emit 796.7 g/kWh, fuel oil-consuming plants –
525 g/kWh, and gas-consuming plants – 377 g/kWh.
Judging by this indicator, solar energy has about 10-
20 times less impact on the environment for the entire
life-cycle period (Greenpeace, 2012). Moreover, as
mentioned above, major emissions occur at the stage
of photovoltaic modules and other SPVP
components’ production (Table 2).
Combustion of hydrocarbon fuel remains the
world leader of harmful emissions into the
atmosphere. Solar energy, in most cases, is perceived
as a technology with practically zero emissions,
which is confirmed by calculations. Some studies also
analyse the effect of SPVPs on harmful emissions to
the atmosphere, throughout the life cycle. They
indicate that throughout SPVP’s entire life-cycle, the
unit emission of greenhouse gas varies from 16 to 86
grams of CO
2
equivalent per kWh generated, while
replacement of fossil fuels can deliver reduction in
emissions from 650 to 850 grams of CO
2
equivalent
per kWh (Ministry of New and Renewable Energy,
2013).
Thus, we conclude that SPVPs have a significant
positive effect on reducing harmful emissions to the
atmosphere, throughout the life cycle of the plant,
while their energy payback indicator is currently the
same as hydrocarbon recovery technologies,
considering their gradual depletion and deterioration
in recoverability.
2.5 Land Use
Depending on the location and type of an SPVP, the
environmental impact, regarding this criterion,
should be assessed in different ways. Thus, large
SPVPs, located on the ground, can cause risk of land
degradation and habitat loss for local fauna. A site
area required varies, depending on the technology,
terrain specific, the site shape, the latitude and other
factors. On average, the required area for an SPVP
placement is 2 to 4 hectares per 1 MW of power.
When placed on artificial surfaces, e.g. on the roof of
a building, an SPVP does not require additional
space.
Land use parameters of SPVPs raise concerns
about the potential impact of such projects on land
and on natural habitats of fauna. This issue is resolved
through regulated examination of each project.
Preference is given to projects that are located on land
plots of various abandoned facilities (airports, mines
etc.). In this case the potential environmental impact
can be significantly reduced and even have a positive
effect of land rehabilitation and economic
reactivation of abandoned land.
Critics of solar energy often claim that SPVPs
require more land than traditional generation
technologies such as coal and natural gas. Similar
criticisms are also put forward against wind farms.
Nevertheless, certain evidence has been published
that large SPVPs in areas with high solar insolation
use less ground than some traditional energy sources,
such as coal generation, considering the use of land
throughout the life cycle of the technology (for
example, the land required for development of coal
mines). Considering the extraction, transportation
and utilization of non-renewable energy sources in
calculating the required land area, SPVPs become
comparable in this regard. At the same time,
installations located on the roof do not take any
additional space at all, while their share in the total
installed capacity of solar energy is more than 80%.
A comparison of the use of land for various
technologies is presented in Table 3.
Traditional energy sources use is often associated
with soil acidification, through precipitation from
hydrogen, sulphates, nitrates of sulphuric acid, nitric
acid and acid rain. Acid precipitation is mainly due to
the combustion of fossil fuels. Rehabilitation of lands
contaminated by acid precipitation or harmful
substances can take decades and, in some cases, even
centuries.
Environmental Characteristics of Solar Photovoltaic Installations Use, Considering Trends of Solar Energy Generation and Sustainable
Development
245