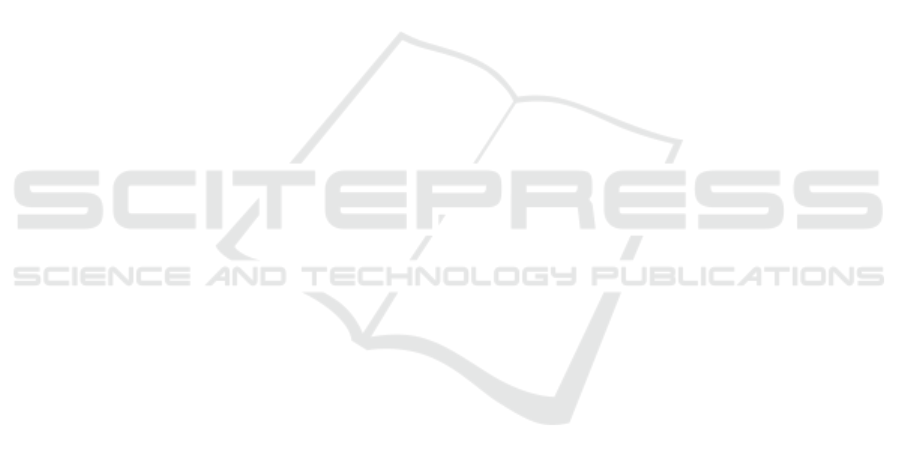
Morphological, Dynamic Mechanical, and Mechanical
Properties of Natural Rubber and Poly (Vinyl Alcohol)
Blends
M Jarnthong
*
, K Chen, R Wang, Y P Cao, F Q Zhang, L S Liao and Z Peng
Key Laboratory of Tropical Crop Products Processing of Ministry of Agriculture,
Agriculture Products Processing Research Institute, Chinese Academy of Tropical
Agricultural Sciences, Zhanjiang 524001, China
Corresponding author and e-mail: M Jarnthong, methakarn.jarnthong@yahoo.com
Abstract. Biopolymer blends of natural rubber (NR) late x and poly (vinyl alcohol) (PVA)
were prepared using the latex blending method. The influence of solid content of NR latex on
morphological, dynamic mechanical, mechanical and thermal p roperties of NR/PVA films
was investigated. The results showed that the solid content of NR latex was consistent with
morphology, dynamic mechanical and mechanical properties of the NR/PVA films.
Reduction of NR particle size in NR/PVA blend, increasing trend o f tensile strength and
elongation at break and changing of glass transition temperature ( T
g
) of PVA phase in the
NR/PVA blend were observed with increasing solid content of NR latex.
1. Introduction
Nowadays, the increasing demand of polymer products increases garbage from their wastes, which is
a widely recognized source of pollution. Since most of thermoplastic and elastic materials do not
decompose easily, elimination of their wastes is a serious environmental concern. To minimize this
problem, many researchers have been developed a new material by blending of conventional polymer
with biodegradable polymer [1, 2]. There are variety of biodegradable polymers obtained from
natural product such as starch, cellulose, chitin and chitosan, from microbial fermentation, such as
polyhydroxybutyrate or obtained by chemical synthesis such as polylactic acid, polycaprolactone,
poly (vinyl alcohol), poly (vinyl chloride) and polysaccharides [3-6]. Among these, poly (vinyl
alcohol) (PVA) is one of the most frequently chosen polymers for biodegradable polymer blend
researches [5, 6]. PVA is a non-toxic water-soluble polymer extensively used in paper coating, textile
sizing, flexible water-soluble packaging films and also in food chemistry, pharmaceuticals and
biomedical applications. PVA could be considered as a good host material in polymer blend due to
good thermo-stability, chemical resistance and film forming ability [7].
Natural rubber (NR) is one of important renewable resource elastomers used in many
manufactures, i.e. rubber tires, gloves, condoms and foam products, due to its good elastic properties,
good resilience and damping behavior. Many researchers have been reported blending of natural
rubber with various types of thermoplastic in molten state to improve mechanical properties of
natural rubber including polystyrene, poly(vinyl chloride) and poly(vinyl alcohol) [8-10].
12
Jarnthong, M., Chen, K., Wang, R., Cao, Y., Zhang, F., Liao, L. and Peng, Z.
Morphological, Dynamic Mechanical, and Mechanical Properties of Natural Rubber and Poly (Vinyl Alcohol) Blends.
In Proceedings of the International Workshop on Materials, Chemistry and Engineering (IWMCE 2018), pages 12-19
ISBN: 978-989-758-346-9
Copyright © 2018 by SCITEPRESS – Science and Technology Publications, Lda. All rights reserved