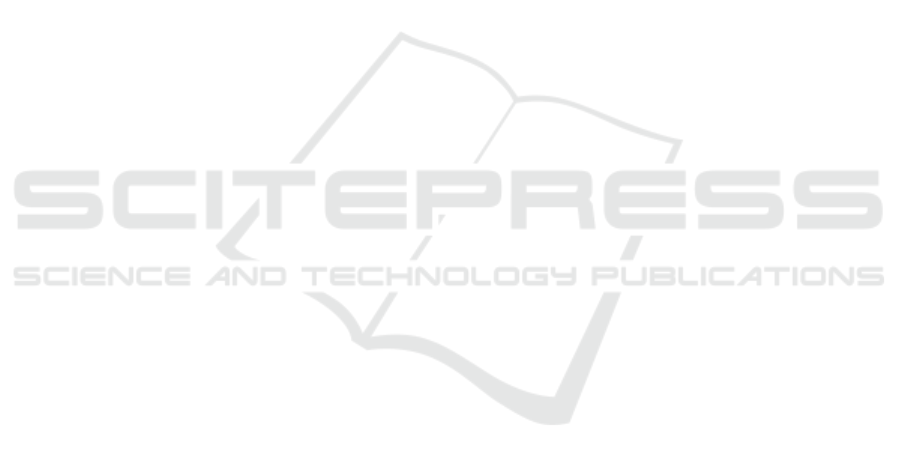
socio-economic and techno-economic approaches can
be developed. While the model is generally applica-
ble to other research projects, it should not be taken as
a guarantee for successful interdisciplinary research,
as this is dependent on multiple factors.
ACKNOWLEDGEMENTS
Special thanks go to the KESS project members for
the fruitful discussions which have contributed to this
paper. Thanks also to Dr. Klaus Baier, Julian Halbey,
Iana Gorokhova and Saskia Ziegler for research sup-
port. The project KESS at RWTH Aachen University
is funded by the strategic funds of the Excellence Ini-
tiative of the German federal and state governments.
REFERENCES
Armstrong, A. and Jackson-Smith, D. (2013). Forms and le-
vels of integration: Evaluation of an interdisciplinary
team-building project. Journal of Research Practice,
9(1):1–20.
Bexten, T., Roscher, B., Weintraub, D., Bachmann, R.,
Schelenz, R., Jacobs, G., Jeschke, P., De Doncker,
R. W., and Wirsum, M. C. (2016a). Modellbasierte
Analyse der Auslegung und des Betriebs kommunaler
Energieversorgungssysteme [Model-based analysis of
the design and operation of municipal energy supply
systems]. In 14. Symposion f
¨
ur Energieinnovation,
EnInnov2016, 10.2.2016-12.02.2016, Graz, Austria.
Bexten, T., Weintraub, D. P., Wirsum, M. C., Roscher, B.,
Bachmann Schiavo, R., Jeschke, P., Jacobs, G., Sche-
lenz, R., and De Doncker, R. W. (2016b). Einfluss be-
trieblicher Flexibilittsparameter einer Gasturbine auf
Auslegung und Betrieb eines dezentralen Energiever-
sorgungssystems [Influence of flexibility parameters
of a gasturbine on design and operation fo a municipal
energy supply system]. In Kraftwerkstechnik 2016:
Strategien, Anlagentechnik und Betrieb, pages 541–
552. Saxonia.
Bexten, T., Wirsum, M., Roscher, B., Schelenz, R., Jacobs,
G., Weintraub, D., Jeschke, P., Bachmann, R., and
De Doncker, R. (2017). Impact of dispatchable energy
conversion and storage units on the electrical autarky
of future municipal energy supply systems. In Procee-
dings of the International Renewable Energy Storage
Conference (IRES) 2017, Dusseldorf, Germany.
Boddington, A., Kermik, J., and Ainsworth, T. (2016). In-
terdisciplinary design in the college of arts and huma-
nities at the university of brighton. In Creating Inno-
vation Leaders, pages 239–254. Springer.
Calero Valdez, A., Schaar, A. K., Ziefle, M., Holzinger, A.,
Jeschke, S., and Brecher, C. (2012). Using mixed node
publication network graphs for analyzing success in
interdisciplinary teams. In International Conference
on Active Media Technology, pages 606–617. Sprin-
ger.
Cummings, J. N. and Kiesler, S. (2005). Collaborative re-
search across disciplinary and organizational bounda-
ries. Social studies of science, 35(5):703–722.
Gross, C. (2007). Community perspectives of wind energy
in Australia: The application of a justice and commu-
nity fairness framework to increase social acceptance.
Energy Policy, 35(5):2727–2736.
Hamann, T., Schaar, A. K., Calero Valdez, A., and Ziefle,
M. (2016). Strategic knowledge management for in-
terdisciplinary teams-overcoming barriers of interdis-
ciplinary work via an online portal approach. In Pro-
ceedings of the International Conference on Human
Interface and the Management of Information, pages
402–413. Springer.
Krohn, W. (2010). Interdisciplinary cases and disciplinary
knowledge. In The Oxford handbook of interdiscipli-
narity, pages 31–49. Oxford University Press.
Lattuca, L. R. (2002). Learning interdisciplinarity: Socio-
cultural perspectives on academic work. The Journal
of Higher Education, 73(6):711–739.
Madlener, R. (2012). Portfolio optimization of power gene-
ration assets. In Handbook of CO in Power Systems,
pages 275–296. Springer.
Marg, S., Hermann, C., Hambauer, V., and Becke, A. B.
(2013). Wenn man was f
¨
ur die Natur machen will,
stellt man da keine Masten hin [If you want to help na-
ture, you don’t build pylons in it]. In Die neue Macht
der B
¨
urger: Was motiviert die Protestbewegungen?
BP-Gesellschaftsstudie, pages 94–138. Rowohlt.
Wilson, G. (2009). The world has problems while univer-
sities have disciplines: Universities meeting the chal-
lenge of environment through interdisciplinary part-
nerships. Journal of the World Universities Forum,
2(2):57–62.
W
¨
ustenhagen, R., Wolsink, M., and B
¨
urer, M. J. (2007).
Social acceptance of renewable energy innovation:
An introduction to the concept. Energy Policy,
35(5):2683–2691.
Zaunbrecher, B. S., Bexten, T., Wirsum, M., and Ziefle, M.
(2016). What is stored, why, and how? Mental mo-
dels, knowledge, and public acceptance of hydrogen
storage. Energy Procedia, 99:108–119.
Zaunbrecher, B. S., Linzenich, A., and Ziefle, M. (2017).
Experts and laypeople’s evaluation of electricity
storage facilities: Implications for communication
strategies. In Proceedings of the International Re-
newable Energy Storage Conference (IRES) 2017,
D
¨
usseldorf, Germany.
Zaunbrecher, B. S. and Ziefle, M. (2016). Integrating
acceptance-relevant factors into wind power planning:
A discussion. Sustainable Cities and Society, 27:307–
314.
Zoellner, J., Schweizer-Ries, P., and Wemheuer, C. (2008).
Public acceptance of renewable energies: Results
from case studies in Germany. Energy Policy,
36(11):4136–4141.
SMARTGREENS 2017 - 6th International Conference on Smart Cities and Green ICT Systems
298