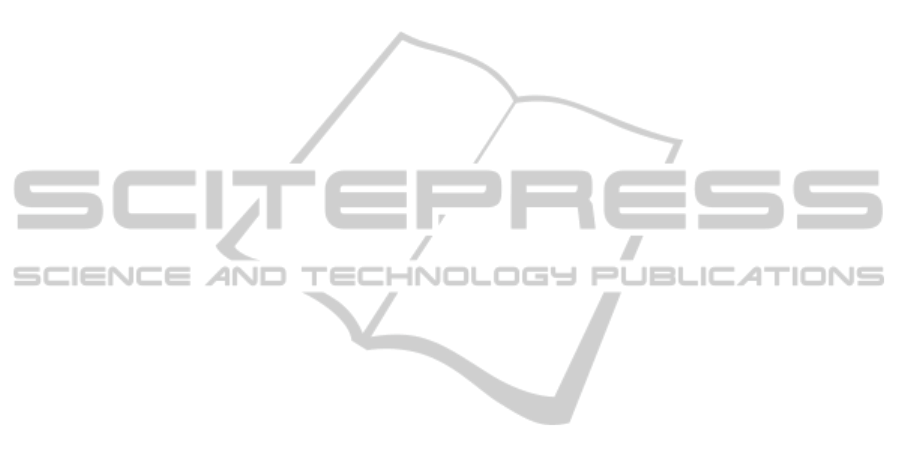
muscle behavior and that it is difficult to maintain
kinesthesia acquired only over a brief period.
In the sets without the light cue in this study,
significantly larger pre-activity was observed before
the onset of MVC. This implies that the muscle was
preparing to establish the timing to exert MVC. It is
known that pre-activity increases so that fatigue-
induced declines in performance do not deteriorate
further (Horita et al., 1999). Therefore, pre-activity
is likely to be a preparatory condition for generating
as large an MVC as possible at an appropriate timing
based solely on kinesthesia, which may explain the
lower self-evaluations of MVC in the 4th set. Pre-
activity gradually decreased after the 4th set and
became closer to the smaller values seen in the 1st to
3rd sets, although the standard deviations of PMT
and PA
5
in the 3rd, 6th, and 9th repetitions were
increased. This suggests a process whereby the
accuracy of movement based on kinesthesia is
decreased due to the disappearance of kinesthetic
information.
Behavior of the muscle fibers during MVC based
solely on kinesthesia was characterized by a large
and unstable PA
5
. The muscle fibers contracted
tightly to a point where the fascicle arises from the
deep aponeurosis, which appeared to compensate for
the delay in the reaction time to start MVC, and
similar muscle fiber behavior has been observed
when the timing to exert MVC was unexpectedly
changed (Hirose et al., 2013). Since muscle force is
transmitted through connective tissues to
neighboring muscles (Huijing, 2003; Sandercock
and Haas, 2009), the behaviour of the muscle fibers
near the deep aponeurosis might result from the
force transmission, and it might be influenced also
by pressure put with a probe fixed over the muscle.
Further studies are needed to elucidate the
mechanism causing strong muscle fiber contractions
near the deep aponeurosis.
Muscle strain injury was reported to occur at or
near the myotendinous junction in frog
myotendinous units when the muscle was strained
(Tidball et al., 1993). Similarly, in human muscles,
muscle failure is created by combining a large force
with substantial stretch near the aponeurosis
(Garrett, 1990), and a clinical study reported most
muscle strain injuries occurring at or near the
myotendinous junction during high-intensity or
explosive voluntary movements (Okuwaki, 2009). If
the muscle fibers are strong and shorten
unexpectedly near the deep aponeurosis with an
inappropriate timing during stretch, the contraction
will increase the inhomogeneous strain on the
aponeurosis (Zuurbier et al., 1994; Kinugasa et al.,
2008), which may cause a muscle strain injury at or
near the myotendinous junction.
In conclusion, behavior of the muscle fiber
during MVC which was exerted based solely on
kinesthesia without the light cue was characterized
by stronger and more unstable contraction near the
deep aponeurosis with longer premotor reaction time
and larger pre-activity. However, the subjects could
not perceive these changes. Such irregular muscle
fiber behavior may be related to a mechanism of
muscle injury.
REFERENCES
Azizi, E., Brainerd, E.L., Roberts, T.J., 2008. Variable
gearing in pinnate muscles. PNAS 105: 1745-1750.
Fukunaga, T., Ichinose, Y., Ito, M., Kawakami, Y.,
Fukashiro, S., 1997. Determination of fascicle length
and pennation in a contracting human muscle in vivo.
J Appl Physiol 82: 354-358.
Garrett, W.E., Jr., 1990. Muscle strain injuries: clinical
and basic aspects. Med Sci Sports Exerc 22: 436-443.
Hawkins, D., Bey, M., 1997. Muscle and tendon force-
length properties and their interactions in vivo. J
Biomech 30: 63-70.
Hayami, T., Kaneko, F., Kizuka, T., 2008. Differences in
the Function of Somatosensory-Motor Integration
depend on the Motor Experiences. SOBIM 19: 47-56.
Hirose, K., Tarodachi, N., Tsutsumi, M., Ogiso, K., 2013.
Timing of muscle contraction influences function of
muscle fibers. In Book of Abstract, 18
th
annual
Congress of the European College of Sport Science.
SporTools.
Horita, T., Komi, P.V., Nicol, C., Kyrolainen, H., 1999.
Effect of exhausting stretch-shortening cycle exercise
on the time course of mechanical behaviour in the
drop jump: possible role of muscle damage. Eur J
Appl Physiol 79: 160-167.
Huijing, PA., 2003. Muscular Force Transmission
Necessitates a Multilevel Integrative Approach to the
Analysis of Function of Skeletal Muscle. Exerc. Sport
Sci. Rev 31(4): 167-175.
Ichinose, Y., Kawakami, Y., Ito, M., Fukunaga, T., 1997.
Estimation of active force-length characteristics of
human vastus lateralis muscle. Acta Anat 159: 78-83.
Kaneko, F., Hayami, T., Yokoi, T., Kizuka, T., 2009.
Research on Examination Indicator of Motor
Performance Corresponding to the Active Kinesthetic
Perception: from the Viewpoint of Detection of
Repetitive Practice Effect. Physical Therapy Japan
36(1): 9-17.
Kinugasa, R., Shin, D., Yamauchi, J., Mishra, C., Hodgson,
J.A., Edgerton, V.R., Sinha, S., 2008. Phase-contrast
MRI reveals mechanical behavior of superficial and
deep aponeuroses in human medial gastrocnemius
during isometric contraction. J Appl Physiol 105:
1312–1320.
MuscleFiberFunctionduringRapidMovementbasedSolelyonKinesthesia
15