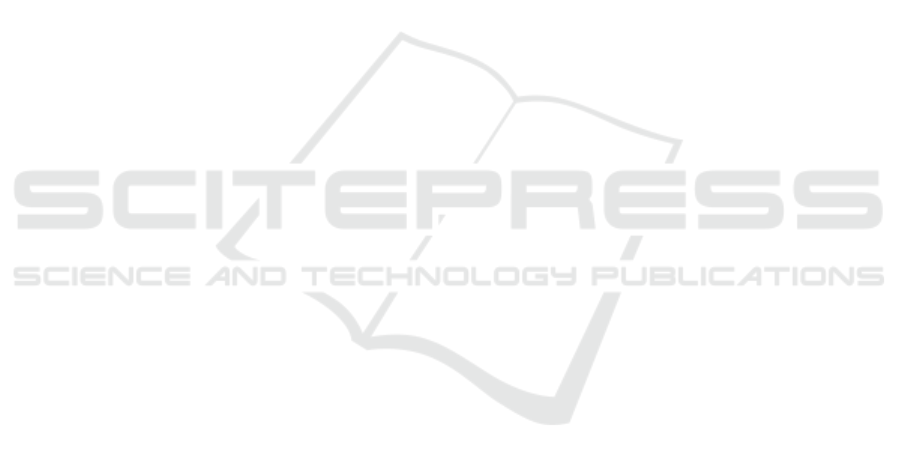
Individual Performance Optimization of Elite Cyclists
Luca Oggiano, Lars Sætran and Lars Morten Bardal
Norwegian University of Science and Technology, Department of Energy and Process Engineering
K. Hejes vei 2b 7042, Trondheim, Norway
Keywords: Aerodynamics, Biomechanics, Wind Tunnel Testing.
Abstract: The present work focuses on individual posture optimization with the aim to individually reduce the drag
and increase the power output on six elite cylists. In order to be able to quantify the changes in drag, power
output and VO2max, wind tunnel tests combined with power output and oxygen intake measurements were
carried out on each of the athletes tested. Drag measurements were performed in the large scale wind tunnel
at NTNU at a constant wind speed of 14.2m/s using a AMTI high frequency force plate. Simultaneously
with the drag measurements, the volume of oxygen intake and the power output generated by the athletes
during the test in different positions were acquired respectively with a Metamax II portable analyzer from
Cortex Biophysic and a Tacx Bushido cycling rig. The main results show that lowering the handlebar while
raising the seat in order to obtain a smaller frontal area and a straighter back, lowers the aerodynamic drag
but will possibly affect the volume of oxygen intake. The handlebar repositioning leaded to similar results
and it might then be questionable whether it is worth reducing the air resistance if the athlete does not sit as
comfortably. In most cases a lower handlebar positioning and a narrower set up of the handlebar resulted in
a considerable drag reduction without compromising the volume of oxygen intake. Being the present work a
preliminary test, no statistical results are presented but as an overall conclusion, it can be pointed out the
need to couple drag force measurements with oxygen intake and power production measurements in order to
have a clearer picture of the effectiveness of the wind tunnel testing.
1 INTRODUCTION
The aerodynamic drag is the main opposing force
that cyclists need to overcome with their generated
power and it counts as 90% ((De Groot et al., 1995,
Di Prampero, 2000, Oggiano et al., 2008)) of the
total forces acting against the athletes motion,
leading to the fact that even small reductions could
then lead to a large improvement in terms of
performances.
(Debraux et al., 2012) gives a clear overview of
the parameters that affect the drag on cyclists and on
the existing methods and theories used to measure it,
minimize it and reduce it, with particular focus on
frontal area direct and indirect measurements and on
frontal area reduction. In his review Debraux also
lists the different methods of assessment of
aerodynamic drag used by different authors dividing
them in wind tunnel tests, linear regression analysis
models, traction resistance measurement methods
and deceleration methods. The author also points at
pros and cons of each measuring method. However,
most methods and tests often focus only on drag (or
frontal area) reduction by modifying and adjusting
the athletes position on the bike, often discarding or
neglecting the side effects that a postural change
might induce in terms of biomechanical and
physiological effectiveness.
A number of authors (Atkinson et al., 2007,
Broker et al., 1999, Di Prampero, 2000, Heil et al.,
2001, Olds, 1998, Olds, 2001, Olds et al., 1995,
Padilla et al., 2000) on the other hand tried to create
mathematical models in order to be able to estimate
the power output generated by the athlete depending
on its posture and on the power required to
overcome the drag and the other resistive forces.
These models often use the posture as input,
modeling the frontal area and the drag and
successively assuming the needed power output.
The present study aims to individually optimize
cyclists performances by simultaneously measuring
oxygen intake, aerodynamic drag and power output
generated in a wind tunnel test. All the adjustments
on the cyclists posture were done keeping the
athletes posture within the parameters stated in the
UCI regulations (UCI, 2012).
79
Oggiano L., Sætran L. and Morten Bardal L..
Individual Performance Optimization of Elite Cyclists.
DOI: 10.5220/0004988700790083
In Proceedings of the 2nd International Congress on Sports Sciences Research and Technology Support (icSPORTS-2014), pages 79-83
ISBN: 978-989-758-057-4
Copyright
c
2014 SCITEPRESS (Science and Technology Publications, Lda.)