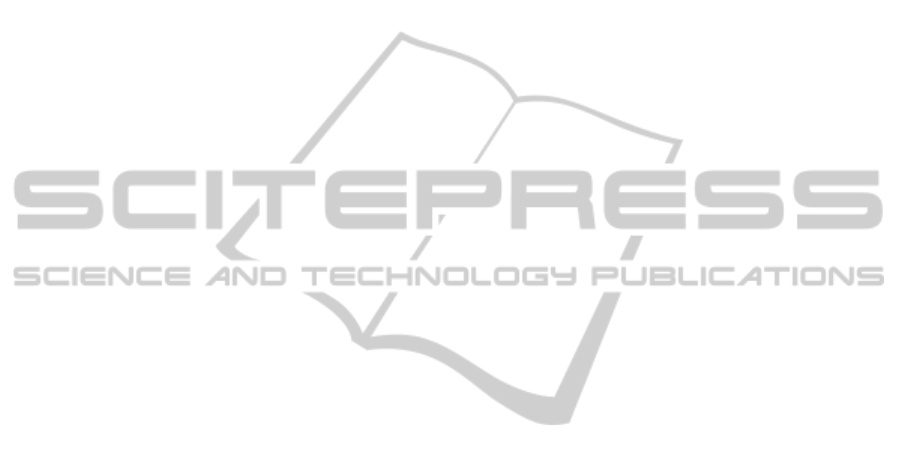
payments according to the expected results.
5 DISCUSSION
Both methods produce results that are differentiated
enough to serve as information basis for regional
and local planning as well as for individual house
owners. In our estimation the solar energy potential
estimation depends on the application of the r.sun
model and on the pvgis data, therefore it can be
applied in every region. However, the accuracy
depends on input data (DEM) and on pvgis data
availability.
For the geothermal energy potential maps we can
consider the typical amount of vertical heat
exchanger per area, which depends on the urban
settlement structure. The necessary input data for
this advanced model are land use maps and average
density of heat exchangers per land use type. With
this approach a comparison based on raster layers
with an energy value per cell would be possible. For
the geothermal energy potential the output energy
for space heating is higher than the energy
abstracted from the ground due to the input of a heat
pump. The seasonal performance factor (spf) of the
heat pump is the dimension of surplus from output
energy dependent of the input energy. The energy
which is available for space heating should be based
on a typical spf factor.
Research projects like Geo-Solar (Pärisch et al.,
2012) and first pilot products from the geothermal
market are focusing of the combination of
solarthermal and geothermal systems. The advantage
of solarthermal systems is the high effectiveness of
collecting energy, the advantage of the geothermal
system is the independent availability of energy 24
hours 365 days a year. Combining both systems
provides a constantly available effective heating
system driven by renewable energy. However, the
potentials of combining both energy sources need
further research. In the future, for a better integration
of both energy sources and the comparison to other
energy forms, all potential maps should be all
transformed to the same ordinal scaling and best
presented on cardinal scales which quantify
kilowatt-hour.
6 CONCLUSION
In many regions geodata already allows the spatially
concrete estimation of solar and geothermal energies
potentials. Our methodologies allow not only for
representing the separate potentials but also give a
first insight into the added value of combining both
sources. The results should be used in regional and
local land use planning as a contribution for
allocating housing development specifically
designed for micro generation of RES. It also can be
used for a targeted and results oriented approach to
allocating incentives to house owners for upgrading
existing houses by RE installation. Last not least the
maps could be made accessible to the public and
used by architects and house owners for calculating
the efficiency of planned solar or geothermal
installations.
ACKNOWLEDGEMENTS
The Lower Saxony research network "Smart Nord"
acknowledges the support of the Lower Saxony
Ministry of Science and Culture through the
"Niedersächsisches Vorab" grant program (grant ZN
2764).
REFERENCES
Baldschuhn, R., Binot, F., Fleig, S. & Kockel, F., 2001.
Geotektonischer Atlas von Nordwestdeutschland und
dem deutschen Nordsee-Sektor: Strukturen,
Strukturentwicklung, Paläogeographie - Geol. Jb.,
A153: 88 p.
Bombien, H., Hoffers, B., Breuckmann, S., Helms, M.,
Lademann, K., Lange, M., Oelrich, A., Reimann, R.,
Rienäcker, J., Schmidt, C., Slaby, M. F. & Ziesch, J.,
2012. Der Geotektonische Atlas von Niedersachsen
und dem deutschen Nordseesektor als geologisches
3D-Modell.- GMIT, 48: 6-13.
EU Joint Research Centre (JRC), 2010. Solar model r.sun.
http://re.jrc.ec.europa.eu/pvgis/solres/solmod3.htm.
LBEG, 2012. Geothermie geht das bei mir.- Online
application, http://nibis.lbeg.de/geothermie/
LBEG, 2013. map server, http://nibis.lbeg.de/cardomap3/
Net!Works European Technology Platform, 2011. Smart
Cities Applications and Requirements, http://www.
networks-etp.eu/fileadmin/user_upload/Publications/P
osition_White_Papers/White_Paper_Smart_Cities_Ap
plications.pdf.
Pannike, S., Kölling, M., Panteleit, B., Reichling, J.,
Scheps, V. & Schulz, H.D., 2006. Auswirkung
hydrogeologischer Kenngrößen auf die Kältefahnen
von Erdwärmesondenanlagen in Lockersedimenten.-
Grundwasser, 1/2006: 6-18.
Pärisch, P., Warmuth, J., Bertram, E., Tepe, R., 2012.
Experiments for combined solar and heat pump
systems. Proceedings of the Eurosun 2012, Rijeka,
Kroatien, 18.-20.09. 2012, Croatian Solar Energy
Association & International Solar Energy Society,
OptimizingMicroRenewableGenerationforSmartCitiesbyCombiningSolarandGeothermalEnergyPotentials-ACase
StudyoftheHannoverRegion
287