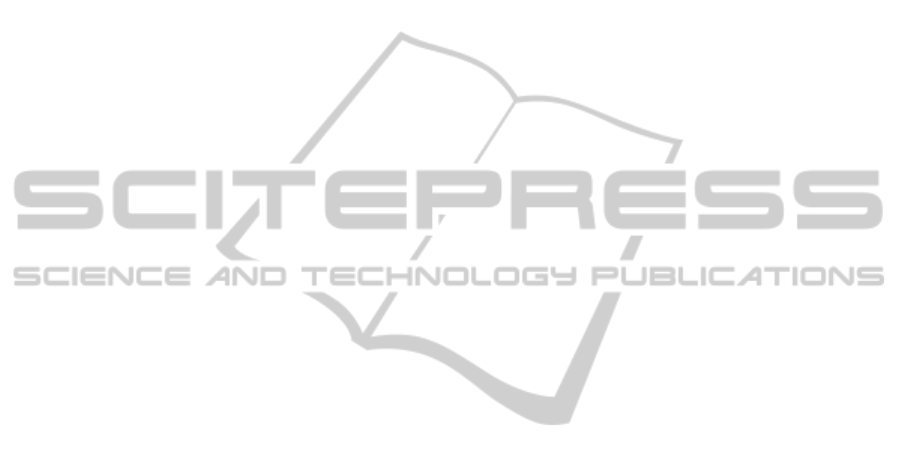
ACKNOWLEDGEMENTS
This study has been supported by a grant from the
Finnish Technology Industries Foundation.
The contribution to LabLife3D by the following
individuals is acknowledged: Päivi Korpelainen,
Elina Kähkönen, Jari Vepsäläinen, Marianne
Hemminki, and Outi Tarakkamäki.
REFERENCES
Arango, F., Chang, C., Esche, S. K. and Chassapis, C.
(2007). A Scenario for Collaborative Learning in
Virtual Engineering Laboratories. In 37th ASEE/IEEE
Frontiers in Education Conference (pp. F3G-7 to
F3G-12). doi:10.1109/FIE.2007.4417818.
Barab, S. A., Hay, K. A., Squire, K., Barnett, K., Schmidt,
R., Karrigan, K., Yamagata-Lynch, L. and Johnson, C.
(2000). Virtual Solar System Project: Learning
Through a Technology-Rich, Inquiry-Based,
Participatory Learning Environment. Journal of
Science Education and Technology, 9, 7–25.
doi:10.1023/A:1009416822783.
Biggs, J. (1999). “What the student does for enhanced
learning”, Higher Education Research and
Development, 18, 57–75.
doi:10.1080/0729436990180105.
Cobb, S., Heaney, R., Corcoran, O. and Henderson-Begg,
S. (2009). The Learning Gains and Student
Perceptions of a Second Life Virtual Lab. Bioscience
Education, 13, article 5. doi:10.3108/beej.13.5.
Daniel, C. (2008). The Educational Attributes of Some of
the World’s “top 50” Universities: A Discussion
Paper. Perth: University of Western Australia.
Dickey, M. D. (2005). Three-Dimensional Virtual Worlds
and Distance Learning: Two Case Studies of Active
Worlds as a Medium for Distance Education. British
Journal of Educational Technology, 36, 439–451.
doi:10.1111/j.1467-8535.2005.00477.x.
Eschenbrenner, B., Nah, F. and Siau, K. (2008). 3-D
virtual worlds in education: Applications, benefits,
issues, and opportunities. Journal of Database
Management, 19, 91–110.
Graves, L. (2008, January 10). A Second Life for Higher
Ed. US News & World Report 14(2), 49–50.
http://www.usnews.com/education/online-education/
articles/2008/01/10/a-second-life-for-higher-ed
(retrieved 9 Nov 2012).
Helmer, J. (2007). Second Life and Virtual Worlds.
No place: Learning Light Limited. http://www.
norfolkelearningforum.co.uk/wp-content/uploads/
2009/04/virtual-worlds_ll_oct_2007.pdf (retrieved 9
Nov 2012).
Holmberg, K. & Huvila, I. (2008). Learning together
apart: Distance education in a virtual world. First
Monday, vol. 13. http://firstmonday.org/htbin/cgiwrap/
bin/ojs/index.php/fm/article/view/2178/2033
(retrieved 9 Nov 2012).
Inman, C., Wright, V. H. and Hartman, J. A. (2010). Use
of Second Life in K-12 and Higher Education: A
Review of Research. Journal of Interactive Online
Learning, 9, 44–63.
http://www.ncolr.org/jiol/issues/pdf/9.1.3.pdf.
Josephsen, J. and Kristensen, A. K. (2006). Simulation of
laboratory assignments to support students’ learning of
introductory inorganic chemistry. Chemistry
Education Research and Practice, 7, 266–279.
doi:10.1039/B6RP90013E.
Kangasniemi, T. (2012). A new environment for chemical
education: The decarboxylation reactions of fatty
acids and experimenting with them in a 3D virtual
laboratory built in Second Life [title from abstract in
English; text is in Finnish]. M.Sc. (Tech.) Thesis.
Espoo: Aalto University School of Chemical
Technology.
Kolmos, A., Du, X., Holgaard, J. and Jensen L.-P. (2008).
Facilitation in a PBL environment. Aalborg: Aalborg
University. ISBN 978-87-991994-8-8.
Mason, H. (2007). Experiential Education in Second Life.
In Proceedings of the Second Life Education
Workshop 2007 (pp. 14–18). http://
www.simteach.com/
slccedu07proceedings.pdf (retrieved 9 Nov 2012).
Mikropoulos, T. A. (2001). Brain Activity on Navigation
in Virtual Environments. Journal of Educational
Computing Research, 24, 1–12.
doi:10.2190/D1W3-Y15D-4UDW-L6C9.
Mikropoulos, T., A. and Natsis, A. (2010). Educational
virtual environments: A ten-year review of empirical
research (1999–2009). Computers & Education, 56,
769–780. doi:10.1016/j.compedu.2010.10.020.
Nielsen, J. (1993). Usability Engineering. Boston, MA:
Academic Press. ISBN 0-12-518406-9.
Nielsen, J. (1994). Heuristic Evaluation. In Nielsen, J. and
Mack, R. L. (eds.), Usability Inspection Methods. New
York, NY: John Wiley & Sons. ISBN 0-471-01877-5.
Nielsen, J. (2005, January 1). 10 Usability Heuristics.
Jakob Nielsen's Alertbox. http://www.nngroup.com/
articles/ten-usability-heuristics/ (retrieved 4 Feb
2013).
Nordström, K., Qvist, P., Natri, O., Närhi, M., Kähkönen,
E., Palomäki, E., Joensuu, P., Jokela, R., Hemminki,
M., Korpelainen, P. and Vepsäläinen, J. (2010).
LabLife3D: A New Concept for Learning and
Teaching Biotechnology and Chemistry in the 21
st
Century Aalto University. In Myller, E. (ed.),
ReflekTori 2010 – Symposium of Engineering
Education, December 9–10, 2010 (pp. 106–108).
Espoo: Aalto University. ISBN 978-952-60-3478-2
(PDF). https://aaltodoc.aalto.fi/bitstream/handle/
123456789/4901/urn100353.pdf (retrieved 9 Nov
2012).
Olkinuora, S. (2012). Design of a Molecular Biology
Laboratory in Second Life. M.Sc. (Tech.) Thesis.
Espoo: Aalto University School of Chemical
Technology.
DesigningVirtualLaboratories-DecarboxylationReactions,VacuumDistillationandVirusIdentificationbyPCRinthe
Lablife3DSecondLifeLaboratory
671