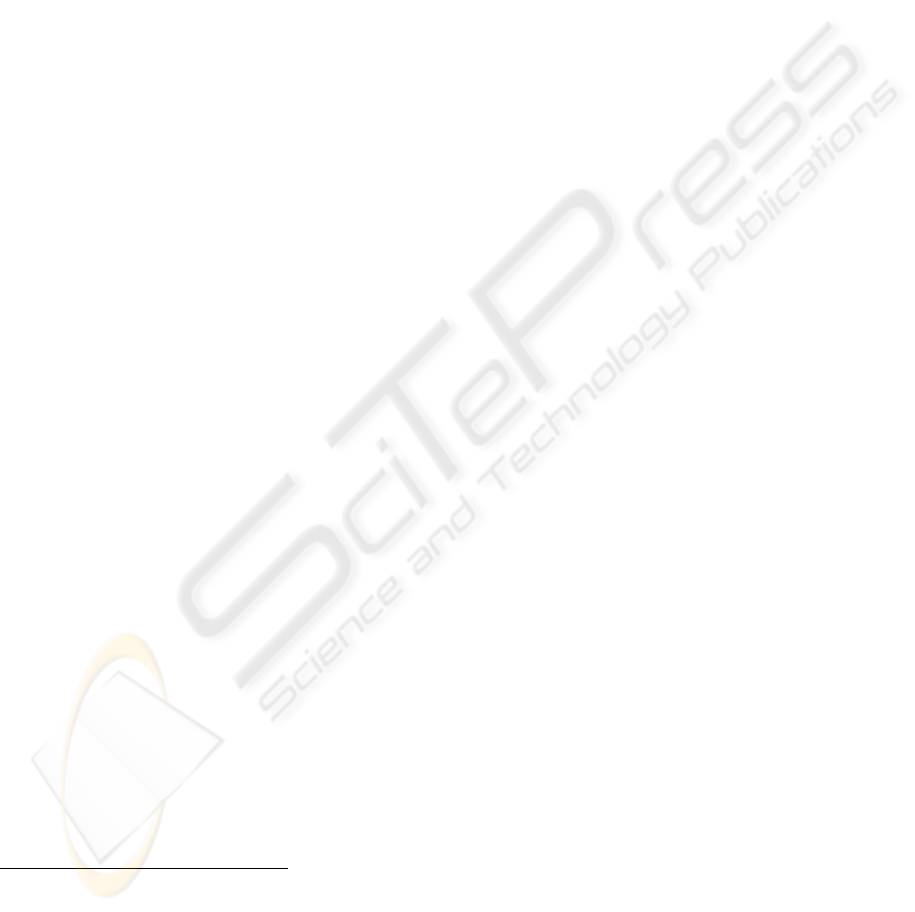
and <.005, respectively. In accordance with the
findings reported by Dori and Belcher (2005), this
study also found that the students in the
low-achieving group benefited most from engaging
in TEAL. Dori and Belcher (2005) stated that it was
probably due to more room for the lower achieving
students to make improvement.
4.2 The Survey Results
A total of 239 surveys were collected from the three
TEAL classes, representing an 85% (239/281) return
rate. The survey information reveals that the TEAL
students were inclined to agree that there was more
interaction taking place between the instructor and
students in the TEAL studio (mean=3.74), when
compared with traditional classroom learning
experiences. They also tended to agree that there
was more interaction taking place among peers in
the TEAL course (mean=4.02). Although they felt
more nervous studying the GP course in the TEAL
studio than in the traditional classroom (mean=3.61),
they, overall, preferred to study in the TEAL studio
than in a traditional classroom.
The three methods most frequently used by the
students to resolve problems encountered were: 1)
discussing the problems with peers, 2) self-studying,
and 3) reviewing the lecture clips posted online. The
top three items (among 14 items
1
) that the students
considered most helpful for their study are: (1) self
study, (2) the instructor’s
instructional style and
teaching skills, and (3) the teaching assistants’
in-class assistance. Suggestions made by the
students for improving future TEAL implementation
revealed that quite a few students were impressed
with the high-technology equipped studio. However,
a number of students stated that the instructional
pace was too fast, formula derivative occurred too
often, and more demonstrations and clearer
explanations of the lecture content were preferred.
5 CONCLUSIONS
Consistent with the findings disclosed in 2007, this
study found that the experimental students not only
1
The 14 items include (1) the instructor’s instructional style and
teaching skills, (2) the use of PRS, (3) attendance requirement (4)
the instructor’s after-class assistance, (5) teaching assistants’
in-class help, (6) recitation sessions taught by teaching assistants,
(7) peers’ in-class discussion, (8) classmates’ after-class assistance,
(9) self study, (10) video clips posted on the web, (11) 3D
simulation, (12) lab activities, (13) frequent tests, and (14)
homework.
showed positive attitudes toward the technology
enabled learning environment, but also achieved
higher learning outcomes than the control students.
This study also disclosed that the learning gain
achieved by the low-achieving TEAL students
(28.14%) was the highest among the groups.
Helping low-achieving students learn more
effectively, and narrowing the learning gap, has been
a common goal for many educators. The results
reported here appear encouraging in this regard.
In addition, the survey results reveal that when
students encountered problems they would discuss
them with their peers in class, which indicates that
the peer-discussion feature of TEAL did provide a
venue for students to solve their problems. However,
it is also noticed that the top three items that the
students considered most helpful to their study were
self-study, the instructor’s instructional skills, and
the teaching assistants’ in-class assistance. In other
words, the students do not yet seem to have fully
benefited from some of the TEAL features, such as
the use of personal response system and simulation
demonstrations. In sum, integrating technological
features into instructional design remains the most
challenging task to the instructors. Nevertheless, it is
hoped that the findings reported here could provide
useful insights and cautions to educators who are
interested in strengthening their teaching through the
use of technology reinforced learning approaches.
NOTE
The research was sponsored by the National Science
Council, NSC 97-2511-S-271-001.
REFERENCES
Bailey, R.C., 1971. Self-concept differences in low and
high achieving students. Journal of Clinical
Psychology, 27(2), 188-191.
Beichner, R., Bernold, L., Burniston, E., Dail, P., Felder,
R., Gastineau, J., Gjersten, M., & Risley, J., 1999.
Case study of the physics components of an integrated
curriculum. American Journal of Physics, 67,
S16-S24.
Beatty, I. D., & Gerace, W.J. (2009). Technology-
enhanced formative assessment: A research-based
pedagogy for teaching science with classroom
response technology. Journal of Science Education
and Technology, 18,146-162.
Beatty, I.D., Leonard, W.J., Gerace, W.J., & Dufresne, R.J.
(2006). Question driven instruction: Teaching science
(well) with an audience response system. In D. A.
CSEDU 2010 - 2nd International Conference on Computer Supported Education
424