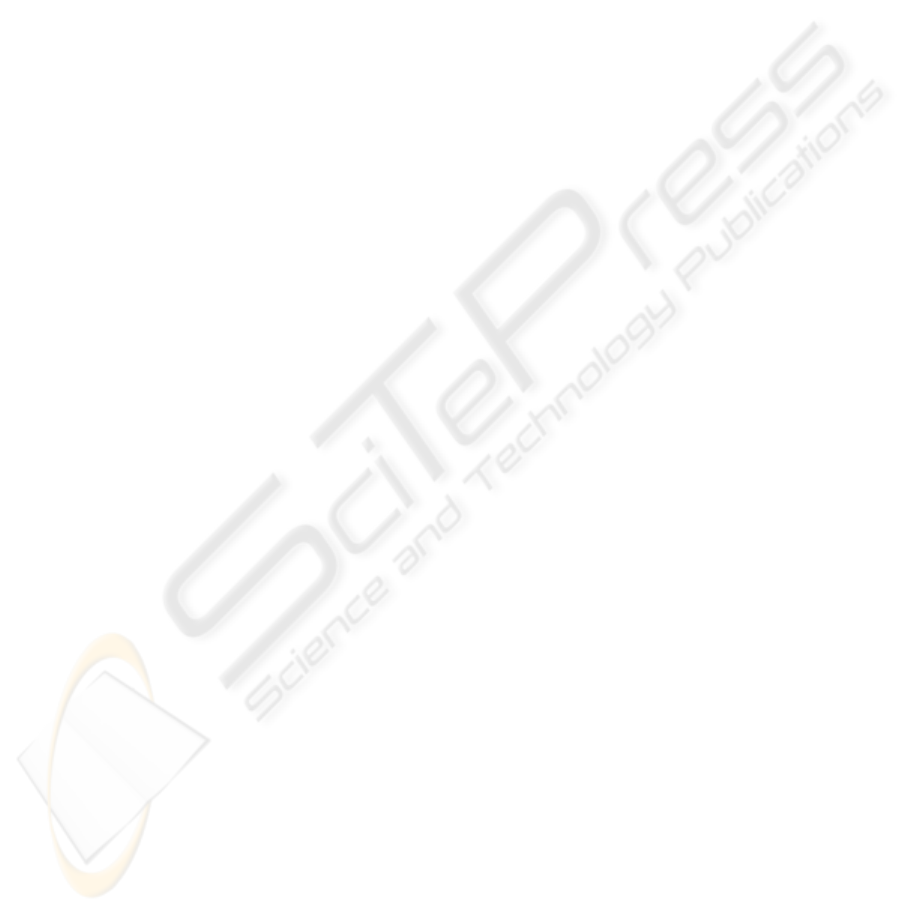
ACKNOWLEDGEMENTS
This work is supported by Grants-in-Aid and
CREST from Japan Science and Technology
Corporation (JST), and Special Coordination Funds
for Promoting Science and Technology of Ministry
of Education, Culture, Sports, Science and
Technology, Japan.
REFERENCES
Arai, K., Tanaka, M., Yamamoto, S., Shimomura, M.,
2008. Colloids Surf A, 313-314, 530.
Cattaneo, E., Mckay, R., 1990. Nature 347, 762.
Louis, S. A., Reynolds, B. A., 2004. Meth. Mole. Biol.
290, 265.
Mcmillan, J. R., Akiyama, M., Tanaka, M., Yamamoto, S.,
Goto, M., Abe, R., Sawamura, D., Shimomura, M,
Shimizu, H., 2007. Tissue Engineering, 13, 789.
Mori, H., Ninomiya, K., Kino-oka, M., Shofuda, T. 2006.
J. Neurosci. Res,. 84, 1682.
Roberti, Y.L., Ronald, D.G., 2000. J. Neurosci. 20, 3725.
Sato, K., Hasebe, K., Tanaka, M., Takebayashi, Nishikawa,
K., Kawai.T., Matsushita, M., Shimomura, M., and S,
Todo., 2002. Inter. J. Nanosci. 1, 689.
Steinman, L., 2003. Nature, 422, 671.
Tanaka, M. Takebayashi, M., Miyama, M., Nishida, J.,
Shimomura, M., 2004. Biomed. Mater. Eng., 14, 439.
Tanaka, M., Nishikawa, K., Okubo, H., Kamachi, H.,
Kawai, T., Matsushita, M., Todo, S., and Shimomura,
M., 2006. Colloids Surf A, 284–285, 464.
Tanaka, M., Takayama, A., Ito, E., Sunami, H.,
Yamamoto, S., Shimomura, M., 2007. J. Nanosci.
Nanotech, 7, 763.
Tanaka, M., Yoshizawa, K., Tsuruma, A., Sunami, H.,
Yamamoto, S., Shimomura, M., 2008. Colloids Surf A,
313-314, 515.
Tsuruma, A., Tanaka, M., Yamamoto, S., Shimomura, M.,
2008. Colloids Surf A, 313-314, 536.
Wang, J. H., Hung, C. H., Young, T. H., 2006.
Biomaterials, 27, 3441.
Wurmser, A. E., Palmer, T. D., Gage, F. H., 2004.
Science, 304, 1253.
Yamamoto, S., Tanaka, M., Sunami, H., Ito, E., Yamashita,
S., Morita, Y., Shimomura, M., 2007. Langmuir, 23,
8114.
CONTROL OF CELL ADHESION AND FUNCTIONS USING SELF-ORGANIZED HONEY COMB-PATTERNED
POLYMER FILMS
393