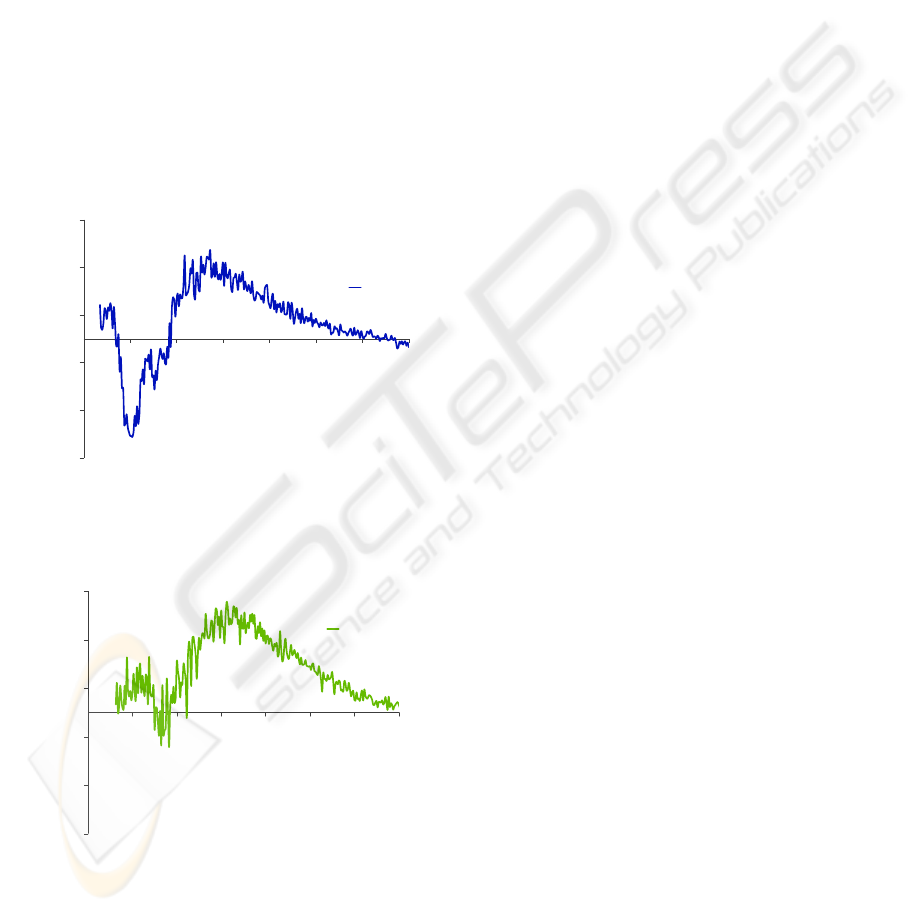
Recent studies have investigated the spectra of
human intestinal mucosa using a spectrofluorometer.
The measurements were performed using short
wavelengths for excitation, and revealed utility in
detecting neoplasia. The AF intensity increased with
neoplasia and had the spectral line shape of
tryptophan, indicating that AF emission from
tryptophan might represent a viable approach to the
detection of malignancy within colonic tissue
(Banerjee, 2000, 2002). A different study had
confirmed that the amount of amino acid related
fluorescence (emission between 300 and 380 nm) is
greater in adenomatous polyps than in the normal
tissues (DaCosta, 2003). Therefore, it can be said
that the results obtained, namely for amino acids AF
emission, are a good initial approach for the design
of optical sensors for the detection of GI early stage
cancer.
‐2.50E+09
‐1.50E+09
‐5.00E+08
5.00E+08
1.50E+09
2.50E+09
360 400 440 480 520 560 600 640
Emissionwavelength(nm)
Autofluorescenceintensity(a.u.)
350nm
Figure 5: Autofluorescence emission spectra of a CACO-2
cell line, with 48 hours growth, obtained for an excitation
wavelength of 350 nm.
‐2.50E+09
‐1.50E+09
‐5.00E+08
5.00E+08
1.50E+09
2.50E+09
345 385 425 465 505 545 585 625
Emission wavelength(nm)
Autofluorescenceintensity(a.u.)
335nm
Figure 6: Autofluorescence emission spectra of a CACO-2
cell line, with 48 hours growth, obtained for an excitation
wavelength of 335 nm.
4 CONCLUSIONS
There is a great interest in developing
autofluorescence-based spectroscopic systems for
the detection of early stage cancers. Understanding
the autofluorescent tissue components and how these
components change in concentration and distribution
with disease is essential in terms of optimizing
diagnostic techniques. Several endogenous
fluorophores have been identified and alterations in
concentrations of these are suggested for
discrimination of normal and malignant tissues.
In this article, it is characterized the AF spectra
of one human GI carcinoma cell line (CACO-2),
using several different excitation wavelengths,
which targeted different molecular species. The
obtained results showed significant AF signal for the
presence of amino acids, namely tryptophan. The
resulting spectral information of the CACO-2 cell
line is consistent with previously published results of
malignant colonic tissues, and is a good initial
approach for the design of fluorescence CMOS
optical sensors, to be incorporated on an EC, for the
differentiation of normal and malignant tissues. It is
important to notice, however, that when comparing
fluorescence spectra of cell monolayers with those
from in vivo or ex vivo tissues is has to be considered
the possible changes due to the physicochemical
microenvironment.
REFERENCES
Banerjee, B., Agarwal, S., Miedema, B., Perez R.,
Chandrasekhar, H. (2000). Use of a shorter
wavelength autofluorescent band to separate
adenomatous from hyperplastic polyps of the colon.
Gastrointestinal Endoscopy, 51, AB149.
Banerjee, B., Chandrasekhar, H. (2002). Use of a single
autofluorescence emission ratio for the detection of
colonic neoplasia. Gastrointestinal Endoscopy, 55,
AB129.
Banerjee, B., Henderson, J., Chaney, T., Davidson, N.
(2004). Detection of Murine Intestinal Adenomas
Using Targeted Molecular Autofluorescence.
Digestive Diseases and Sciences, 49, 54-59.
DaCosta, R. S., Wilson, B. C., Marcon, N. E. (2002). New
optical technologies for earlier endoscopic diagnosis
of premalignant gastrointestinal lesions. Journal of
Gastroenterology and Hepatology, 17, S85-S104.
DaCosta, R. S., Andersson, H., Wilson, B. C. (2003).
Molecular Fluorescence Excitation–Emission Matrices
Relevant to Tissue Spectroscopy. Photochemistry and
Photobiology, 78, 384-392.
DaCosta, R. S., Andersson, H., Cirocco, M., Marcon, N.
E., Wilson, B. C. (2005). Autofluorescence
AUTOFLUORESCENCE SPECTROSCOPY OF A HUMAN GASTROINTESTINAL CARCINOMA CELL LINE -
Design of Optical Sensors for the Detection of Early Stage Cancer
65