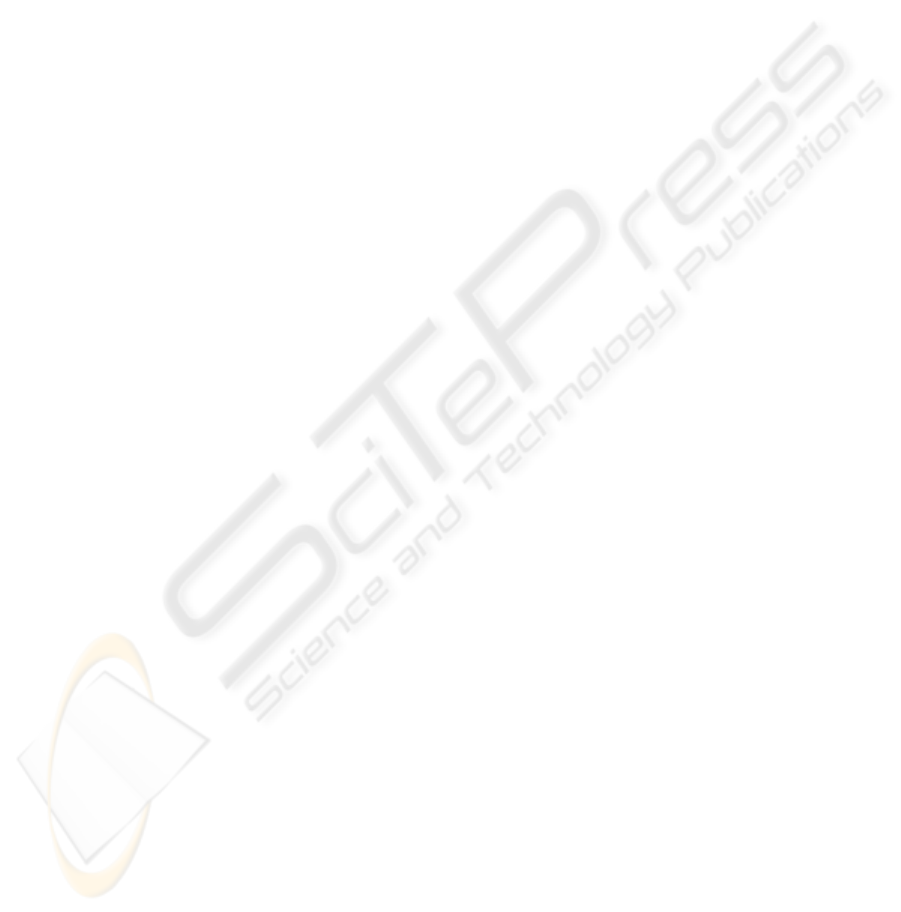
The authors are convinced that these first
promising results are one step closer to a new era of
surgical instruments giving "reality-like feelings" in
endoscopic and teleoperated surgery.
ACKNOWLEDGEMENTS
The work described in this paper was supported by
the ASSEMIC project, a Marie Curie Research &
Training Network (MRTN-CT- 2003-504826) and
PROFACTOR Research and Solutions GmbH –
Austria. A special mention to Mr. C. Filippeschi for
his continuous and invaluable help.
REFERENCES
Bholat, O. S. , Haluck, R. S., Murray, W. B., Gorman, P.
J. and Krummel, T. M., 1999, “Tactile feedback is
present during minimally invasive surgery”, J Am
Coll Surg,, 189(4):349–55.
Cavusoglu, M. C., Williams, W., Tendick, F. and Sastry,
S.S., 2001 “Robotics for telesurgery: second
generation Berkeley/UCSF laparoscopic telesurgical
workstation and looking towards the future
applications” In Proceedings of .the 39th Allerton
Conference of Communication, Control and
Computing.
Guthart, G. S. and Salisbury, J. K., 2000 “The Intuitive
TM
telesurgery system: overview and application”, in
Proceedings of ICRA 2000, pages 618–621, DaVinci.
Wagner,C.R., Perrin,D.P., Howe,R.D., “Force Feedback
in a Three-Dimensional Ultrasound-Guided Surgical
Task”, Symposium on Haptic Interfaces for Virtual
Environment and Teleoperator Systems 2006, March
25 - 26, Alexandria, Virginia, USA
Pillarisetti,A.,Pekarev,M., Brooks,A.D., Desai, J.P.,
“Evaluating the Role of Force Feedback for
Biomanipulation Tasks” Symposium on Haptic
Interfaces for Virtual Environment and Teleoperator
Systems 2006 March 25 - 26, Alexandria, Virginia,
USA
Pitakwatchara,P., Warisawa,S.,Mitsuishi, M., “Force
Feedback Augmentation Modes in the Laparoscopic
Minimal Invasive Telesurgical System” Aug. 2007,
IEEE /ASME Transactions on Mechatronics Volume:
12, Issue: 4, pages: 447-454
Kazi, A., 2001, “Operator performance in surgical
telemanipulation. Presence”, 10:495– 510.
Kitagawa, M., Okamura, A.M., Bethea, B.T., Gott, V.L.,
and Baumgartner, A.A., “Analysis of suture
manipulation forces for teleoperation with force
feedback”, /Lect. Not. Comp. Sci./, vol. 2488, pp.
155–162.
Mayer, H., N´agy, I., Knoll, A., Schirmbeck E. U., and
Bauernschmitt, R., 2005 “An experimental system for
robotic heart surgery” in IEEE 18th International
Symposium on Computer-Based Medical Systems
(CBMS), Dublin, Ireland.
Menciassi, A., Eisinberg, A., Carrozza, M.C. and Dario.
P., 2003, “Force sensing microinstrument for
measuring tissue properties and pulse in
microsurgery”, IEEE/ASME Transactions on
Mechatronics, 8(1):10–17.
Menciassi, A., Eisinberg, A., Scalari, G., Anticoli, C.,
Carrozza,M.C. and Dario, P., 2001 ,“Force feedback-
based microinstrument for measuring tissue properties
and pulse in microsurgery”, in Proc. IEEE
International Conference on Robotics and Automation
(ICRA).
Rosen, J., Hannaford, B., MacFarlane, M., and Sinanan,
M., 1999 “Force controlled and teleoperated
endoscopic grasper for minimally invasive surgery –
experimental performance evaluation”, IEEE
Transactions on Biomedical Engineering,
46(10):1212–1221.
Sheridan, T.B., 1992, “Telerobotics, automation and
human supervisory control”, MIT Press, Cambridge,
MA, USA.
Tavakoli, M., Patel, R.V. and Moallem, M. , 2005 ,
“Haptic interaction in robot-assisted endoscopic
surgery: a sensorized end-effector”, International
Journal of Medical Robotics and Computer Assisted
Surgery, 1(2):53–63.
Houston, K., Eder, C., Sieber, A., Menciassi, A. Carrozza,
M. C., Dario, P., 2007 “Polymer sensorised
microgrippers using SMA actuation”, ICRA 2007.
Eisinberg, A., Izzo, I., Menciassi, A. Houston, K. ,
Valdastri, P., Dario, P., Gustafsson., R., Simu, U.,
Johansson S., 2005 , “Design and shape deposition
manufacturing (SDM) fabrication of a PZT-actuated
tool for micromanipulation”, Computational
Intelligence in Robotics and Automation, CIRA 2005.
Harada, K., Tsubouchi, K., Fujie, M. G., Chiba, T., 2005,
“Micro Manipulators for Intrauterine Fetal Surgery in
an Open MRI”, Proceedings of the 2005 IEEE
International Conference on Robotics and Automation
Barcelona, Spain.
NOVEL HAPTIC TOOL AND INPUT DEVICE FOR BILATERAL BIOMANIPULATION ADDRESSING
ENDOSCOPIC SURGERY
69