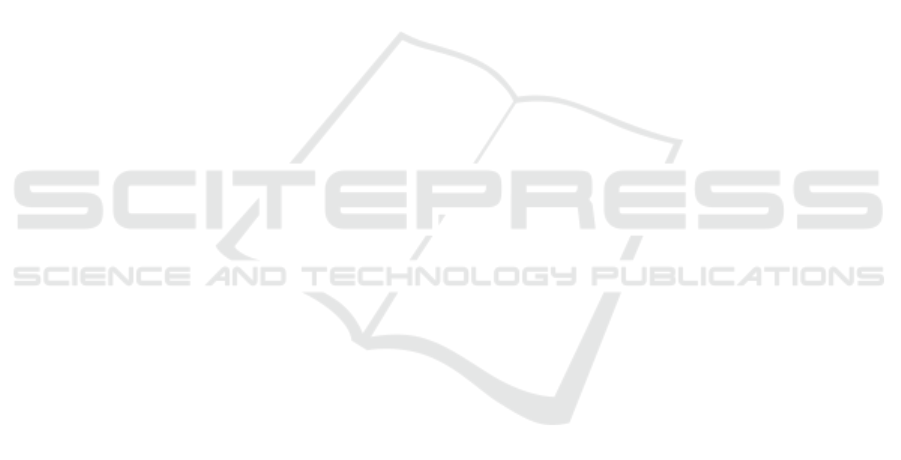
Iacono, M. and Sgorbissa, A. (2018). Path following and
obstacle avoidance for an autonomous uav using a
depth camera. Robotics and Autonomous Systems,
106:38–46.
Jia, Y., Guo, L., and Wang, X. (2018). Real-time control
systems. In Transportation Cyber-Physical Systems,
pages 81–113. Elsevier.
Kato, N., Kawamoto, Y., Aneha, A., Yaguchi, Y., Miura, R.,
Nakamura, H., Kobayashi, M., Henmi, T., Akimoto,
O., Kamisawa, Y., et al. (2019). Location awareness
system for drones flying beyond visual line of sight
exploiting the 400 mhz frequency band. IEEE Wire-
less Communications, 26(6):149–155.
Kodheli, O., Guidotti, A., and Vanelli-Coralli, A. (2017).
Integration of satellites in 5g through leo constella-
tions. In GLOBECOM 2017-2017 IEEE Global Com-
munications Conference, pages 1–6. IEEE.
Kovalev, I., Voroshilova, A., and Karaseva, M. (2019).
Analysis of the current situation and development
trend of the international cargo uavs market. In Jour-
nal of Physics: Conference Series, volume 1399, page
055095. IOP Publishing.
Krishnan, P. and Manimala, K. (2020). Implementation of
optimized dynamic trajectory modification algorithm
to avoid obstacles for secure navigation of UAV. Ap-
plied Soft Computing, 90:106168.
Kyrkou, C., Timotheou, S., Kolios, P., Theocharides, T., and
Panayiotou, C. (2019). Drones: augmenting our qual-
ity of life. IEEE Potentials, 38(1):30–36.
Lin, C., He, D., Kumar, N., Choo, K.-K. R., Vinel, A., and
Huang, X. (2018). Security and privacy for the inter-
net of drones: Challenges and solutions. IEEE Com-
munications Magazine, 56(1):64–69.
Macias, M., Barrado, C., Pastor, E., and Royo, P. (2019).
The future of drones and their public acceptance. In
2019 IEEE/AIAA 38th Digital Avionics Systems Con-
ference (DASC), pages 1–8. IEEE.
Merkert, R. and Bushell, J. (2020). Managing the drone rev-
olution: A systematic literature review into the current
use of airborne drones and future strategic directions
for their effective control. Journal of Air Transport
Management, 89:101929.
Mohamed, N., Al-Jaroodi, J., Jawhar, I., Idries, A., and Mo-
hammed, F. (2020). Unmanned aerial vehicles appli-
cations in future smart cities. Technological Forecast-
ing and Social Change, 153:119293.
Mousavi, S., Afghah, F., Ashdown, J. D., and Turck, K.
(2019). Use of a quantum genetic algorithm for coali-
tion formation in large-scale uav networks. Ad Hoc
Networks, 87:26–36.
Nguyen, H. C., Amorim, R., Wigard, J., Kovacs, I. Z.,
and Mogensen, P. (2017). Using lte networks for
UAV command and control link: A rural-area cover-
age analysis. In 2017 IEEE 86th Vehicular Technology
Conference (VTC-Fall), pages 1–6. IEEE.
Nouacer, R., Hussein, M., Espinoza, H., Ouhammou, Y.,
Ladeira, M., and Castiñeira, R. (2020). Towards a
framework of key technologies for drones. Micropro-
cessors and Microsystems, page 103142.
Otto, A., Agatz, N., Campbell, J., Golden, B., and Pesch, E.
(2018). Optimization approaches for civil applications
of unmanned aerial vehicles (uavs) or aerial drones: A
survey. Networks, 72(4):411–458.
Outay, F., Mengash, H. A., and Adnan, M. (2020). Applica-
tions of unmanned aerial vehicle (uav) in road safety,
traffic and highway infrastructure management: Re-
cent advances and challenges. Transportation re-
search part A: policy and practice, 141:116–129.
Rakha, T. and Gorodetsky, A. (2018). Review of unmanned
aerial system (UAS) applications in the built environ-
ment: Towards automated building inspection pro-
cedures using drones. Automation in Construction,
93:252–264.
Santoso, F., Garratt, M. A., and Anavatti, S. G. (2017).
State-of-the-art intelligent flight control systems in
unmanned aerial vehicles. IEEE Transactions on Au-
tomation Science and Engineering, 15(2):613–627.
Sebbane, Y. B. (2018). Intelligent autonomy of UAVs: ad-
vanced missions and future use. CRC Press.
Undertaking, S. J. (2018). European atm master plan:
Roadmap for the safe integration of drones into all
classes of airspace. SESAR Joint Undertaking: Brus-
sels, Belgium.
van der Merwe, D., Burchfield, D. R., Witt, T. D., Price,
K. P., Sharda, A., M ˛acik, M., Gryta, A., Fr ˛ac, M.,
Dwivedi, S. L., Goldman, I., et al. (2020). Drones
in agriculture. Advances in Agronomy, 160:1.
Wang, C., Wang, J., Shen, Y., and Zhang, X. (2019).
Autonomous navigation of uavs in large-scale com-
plex environments: A deep reinforcement learning ap-
proach. IEEE Transactions on Vehicular Technology,
68(3):2124–2136.
Wang, J., Jiang, C., Han, Z., Ren, Y., Maunder, R. G., and
Hanzo, L. (2017). Taking drones to the next level: Co-
operative distributed unmanned-aerial-vehicular net-
works for small and mini drones. Ieee vehIcular tech-
nology magazIne, 12(3):73–82.
Wang, X., Hu, J., and Lin, H. (2020). An intelligent uav
based data aggregation strategy for iot after disaster
scenarios. In Proceedings of the 2nd ACM MobiCom
Workshop on Drone Assisted Wireless Communica-
tions for 5G and Beyond, pages 97–101.
Webster, M., Western, D., Araiza-Illan, D., Dixon, C., Eder,
K., Fisher, M., and Pipe, A. G. (2020). A corrobora-
tive approach to verification and validation of human–
robot teams. The International Journal of Robotics
Research, 39(1):73–99.
Xie, Y., Savvaris, A., and Tsourdos, A. (2019). Fuzzy logic
based equivalent consumption optimization of a hy-
brid electric propulsion system for unmanned aerial
vehicles. Aerospace Science and Technology, 85:13–
23.
Xu, X., Wang, T., Lu, Q., and Shi, Y. (2018). Resource con-
strained cellular neural networks for real-time obsta-
cle detection using FPGAs. In 2018 19th International
Symposium on Quality Electronic Design (ISQED),
pages 437–440. IEEE.
VEHITS 2021 - 7th International Conference on Vehicle Technology and Intelligent Transport Systems
512