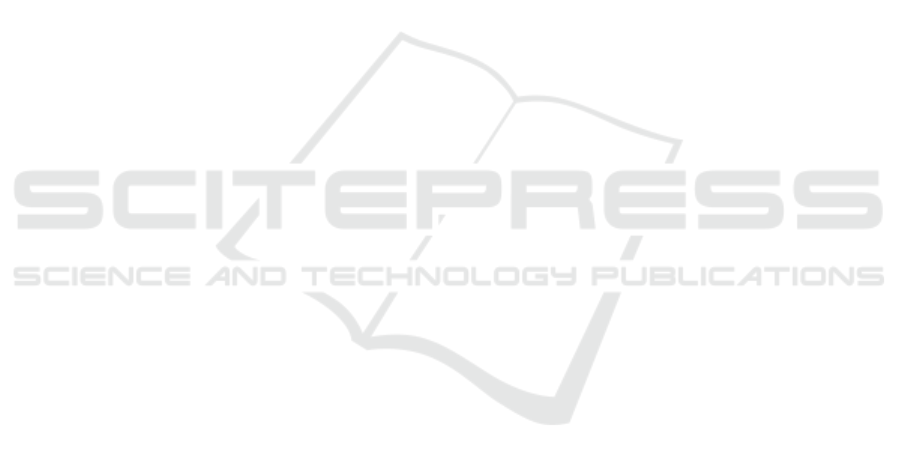
effect during changes in left ventricular volume. The
current design is limited by the use of the right leg
of the animal as ground for the data acquisition soft-
ware. Furthermore, the current design of the unipolar
amplifier does not actively isolate the subject from the
DAQ. The performance of a fully portable system will
need to be re-evaluated using a similar experimental
setup as proposed in this paper.
ACKNOWLEDGEMENTS
The authors have no conflict of interest relevant to this
publication. The authors thankfully acknowledge the
financial support by the Georg und Bertha Schwyzer-
Winiker Foundation, the IMG Foundation, as well as
the ETH Zurich Foundation. This work is part of the
Zurich Heart project under the umbrella of Univer-
sity Medicine Zurich. Furthermore,the authors thank
Simon Suendermann, Christoph Starck, Nikola Ce-
sarovic, Mareike Kron and Marko Canic for their sup-
port with the animal study and Sara Mettler for the
electrical engineering support.
REFERENCES
Amoore, J. N. (1985). The brody effect and change of
volume of the heart. Journal of Electrocardiology,
18(1):71–75.
Battler, A., Froelicher, V. F., Gallagher, K. P., Kumada, T.,
McKown, D., Kemper, W. S., and Ross, J. (1980). Ef-
fects of changes in ventricular size on regional and
surface QRS amplitudes in the conscious dog. Circu-
lation, 62(1):174–180.
Brody, D. A. (1956). A Theoretical Analysis of Intracav-
itary Blood Mass Influence on the Heart-Lead Rela-
tionship. Circulation Research, IV:731–737.
Cysyk, J., Newswanger, R., Popjes, E., Pae, W., Jhun, C.-
S., Izer, J., Weiss, W., and Rosenberg, G. (2018).
Cannula Tip With Integrated Volume Sensor for Ro-
tary Blood Pump Control: Early-Stage Development.
ASAIO journal.
Dual, S. A., Ochsner, G., Petrou, A., Amacher, R., Wilhelm,
M., Meboldt, M., and Schmid Daners, M. (2016). R-
Wave Magnitude : a Control Input for Ventricular As-
sist Devices. International Workshop on Biosignal In-
terpretation, Osaka.
Dual, S. A., Zimmermann, J. M., Neuenschwander, J.,
Cohrs, N. H., Solowjowa, N., Stark, W. J., Meboldt,
M., and Schmid Daners, M. (2019). Ultrasonic sensor
concept to fit a ventricular assist device cannula evalu-
ated using geometrically accurate heart phantoms. Ar-
tificial Organs, 43(5):467–477.
Gargiulo, G. D. (2015). True Unipolar ECG Machine for
Wilson Central Terminal Measurements. BioMed Re-
search International, 2015.
Gargiulo, G. D., McEwan, A. L., Bifulco, P., Cesarelli, M.,
Jin, C., Tapson, J., Thiagalingam, A., and Van Schaik,
A. (2013). Towards true unipolar bio-potential record-
ing: A preliminary result for ECG. Physiological
Measurement, 34(1).
Haines, D. E., Wong, W., Canby, R., Jewell, C., Houmsse,
M., Pederson, D., Sugeng, L., Porterfield, J., Kottam,
A., Pearce, J., Valvano, J., Michalek, J., Trevino, A.,
Sagar, S., and Feldman, M. D. (2017). Validation
of a defibrillation lead ventricular volume measure-
ment compared to three-dimensional echocardiogra-
phy. Heart Rhythm, 14(10):1515–1522.
Kirklin, J. K., Xie, R., Cowger, J., de By, T. M., Nakatani,
T., Schueler, S., Taylor, R., Lannon, J., Mohacsi,
P., Gummert, J., Goldstein, D., Caliskan, K., and
Hannan, M. M. (2018). Second annual report from
the ISHLT Mechanically Assisted Circulatory Support
Registry. The Journal of Heart and Lung Transplan-
tation, 37(6):685–691.
Madias, J. E., Song, J., White, C. M., Kalus, J. S., and
Kluger, J. (2005). Response of the ECG to Short-Term
Diuresis in Patients with Heart Failure. Annals of Non-
invasive Electrocardiology, 10(3):288–296.
Ochsner, G., Amacher, R., Wilhelm, M. J., Vandenberghe,
S., Tevaearai, H., Plass, A., Amstutz, A., Falk, V.,
and Schmid Daners, M. (2014). A Physiological Con-
troller for Turbodynamic Ventricular Assist Devices
Based on a Measurement of the Left Ventricular Vol-
ume. Artificial organs, 38(7):527–538.
Ochsner, G., Wilhelm, M. J., Amacher, R., Petrou, A., Ce-
sarovic, N., Staufert, S., R
¨
ohrnbauer, B., Maisano, F.,
Hierold, C., Meboldt, M., and Schmid Daners, M.
(2017). In Vivo Evaluation of Physiologic Control Al-
gorithms for Left Ventricular Assist Devices Based on
Left Ventricular Volume or Pressure. ASAIO Journal,
63(5):568–577.
Petrou, A., Lee, J., Dual, S., Ochsner, G., Meboldt, M.,
and Schmid Daners, M. (2018). Standardized Com-
parison of Selected Physiological Controllers for Ro-
tary Blood Pumps: In Vitro Study. Artificial Organs,
42(3):E29–E42.
Schima, H., Trubel, W., Moritz, A., Wieselthaler, G., Stohr,
H. G., Thoma, H., Losert, U., and Wolner, E. (2008).
Noninvasive Monitoring of Rotary Blood Pumps: Ne-
cessity, Possibilities, and Limitations. Artificial Or-
gans, 16(2):195–202.
van Oosterom, A. (2010). Macroscopic Source Descrip-
tions. In Macfarlane, P., van Oosterom, A., Pahlm,
O., Kligfield, P., Janse, M., and Camm, J., edi-
tors, Comprehensive Electrocardiology, pages 193–
225. Springer Verlag, London, 2011 edition.
BIODEVICES 2021 - 14th International Conference on Biomedical Electronics and Devices
48