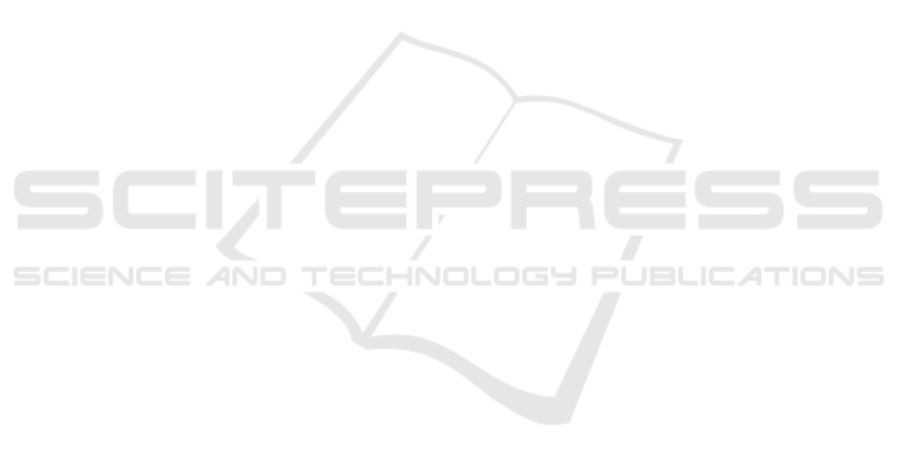
and diabetes. American Journal of Health Behavior,
40(2):194–204.
Borges, U., Laborde, S., and Raab, M. (2019). Influ-
ence of transcutaneous vagus nerve stimulation on car-
diac vagal activity: Not different from sham stimula-
tion and no effect of stimulation intensity. PloS one,
14(10):e0223848.
Constantinescu, V., Matei, D., Constantinescu, I., and Cuci-
ureanu, D. I. (2019). Heart rate variability and vagus
nerve stimulation in epilepsy patients. Translational
Neuroscience, 10(1):223–232.
De Couck, M., Cserjesi, R., Caers, R., Zijlstra, W., Widjaja,
D., Wolf, N., Luminet, O., Ellrich, J., and Gidron,
Y. (2017). Effects of short and prolonged transcuta-
neous vagus nerve stimulation on heart rate variability
in healthy subjects. Autonomic Neuroscience, 203:88–
96.
Dietrich, S., Smith, J., Scherzinger, C., Hofmann-Preiß, K.,
Freitag, T., Eisenkolb, A., and Ringler, R. (2008). A
novel transcutaneous vagus nerve stimulation leads to
brainstem and cerebral activations measured by func-
tional mri. Biomedizinische Technik/Biomedical Engi-
neering, 53(3):104–111.
Fang, J., Rong, P., Hong, Y., Fan, Y., Liu, J., Wang, H.,
Zhang, G., Chen, X., Shi, S., and Wang, L. (2016).
Transcutaneous vagus nerve stimulation modulates
default mode network in major depressive disorder.
Biological Psychiatry, 79(4):266–273.
Hein, E., Nowak, M., Kiess, O., Biermann, T., Bayerlein,
K., Kornhuber, J., and Kraus, T. (2013). Auricu-
lar transcutaneous electrical nerve stimulation in de-
pressed patients: a randomized controlled pilot study.
Journal of Neural Transmission, 120(5):821–827.
Hidaka, B. H. (2012). Depression as a disease of moder-
nity: Explanations for increasing prevalence. Journal
of Affective Disorders, 140(3):205 – 214.
Johnson, R. L. and Wilson, C. G. (2018). A review of vagus
nerve stimulation as a therapeutic intervention. Jour-
nal of Inflammation Research, 11:203.
Kamath, M., Upton, A., Talalla, A., and Fallen, E. (1992).
Effect of vagal nerve electrostimulation on the power
spectrum of heart rate variability in man. Pacing and
Clinical Electrophysiology, 15(2):235–243.
Kaniusas, E., Kampusch, S., Tittgemeyer, M., Panetsos, F.,
Gines, R. F., Papa, M., Kiss, A., Podesser, B., Cas-
sara, A. M., Tanghe, E., Samoudi, A. M., Tarnaud, T.,
Joseph, W., Marozas, V., Lukosevicius, A., I
ˇ
stuk, N.,
ˇ
Saroli
´
c, A., Lechner, S., Klonowski, W., Varoneckas,
G., and Sz
´
eles, J. C. (2019a). Current directions in the
auricular vagus nerve stimulation i – a physiological
perspective. Frontiers in Neuroscience, 13(854).
Kaniusas, E., Kampusch, S., Tittgemeyer, M., Panetsos, F.,
Gines, R. F., Papa, M., Kiss, A., Podesser, B., Cas-
sara, A. M., Tanghe, E., Samoudi, A. M., Tarnaud, T.,
Joseph, W., Marozas, V., Lukosevicius, A., I
ˇ
stuk, N.,
Lechner, S., Klonowski, W., Varoneckas, G., Sz
´
eles,
J. C., and
ˇ
Saroli
´
c, A. (2019b). Current directions in
the auricular vagus nerve stimulation ii – an engineer-
ing perspective. Frontiers in Neuroscience, 13(772).
Kessler, R. C., Ruscio, A. M., Shear, K., and Wittchen, H.-
U. (2009). Epidemiology of anxiety disorders. In Be-
havioral Neurobiology of Anxiety and Its Treatment,
pages 21–35. Springer.
Kim, H.-G., Cheon, E.-J., Bai, D.-S., Lee, Y. H., and Koo,
B.-H. (2018). Stress and heart rate variability: a meta-
analysis and review of the literature. Psychiatry inves-
tigation, 15(3):235.
Malik, M., Bigger, J. T., Camm, A. J., Kleiger, R. E.,
Malliani, A., Moss, A. J., and Schwartz, P. J. (1996).
Heart rate variability: Standards of measurement,
physiological interpretation, and clinical use. Euro-
pean heart journal, 17(3):354–381.
McClintock, S. M., Trevino, K., and Husain, M. M. (2009).
Vagus nerve stimulation: Indications, efficacy, and
methods. In Swartz, C. M., editor, Electroconvul-
sive and Neuromodulation Therapies, pages 543–555.
Cambridge University Press.
Mouli, S. and Palaniappan, R. (2017). Toward a reliable
pwm-based light-emitting diode visual stimulus for
improved ssvep response with minimal visual fatigue.
The Journal of Engineering, 2017(2):7–12.
Mouli, S. and Palaniappan, R. (2020). DIY hybrid SSVEP-
P300 LED stimuli for BCI platform using EMOTIV
EEG headset. HardwareX, 8:e00113.
Rong, P., Liu, J., Wang, L., Liu, R., Fang, J., Zhao, J., Zhao,
Y., Wang, H., Vangel, M., and Sun, S. (2016). Ef-
fect of transcutaneous auricular vagus nerve stimula-
tion on major depressive disorder: a nonrandomized
controlled pilot study. Journal of Affective Disorders,
195:172–179.
Sclocco, R., Garcia, R. G., Gabriel, A., Kettner, N. W., Na-
padow, V., and Barbieri, R. (2017). Respiratory-gated
auricular vagal afferent nerve stimulation (ravans) ef-
fects on autonomic outflow in hypertension. In 2017
39th Annual Inter. Conf. of the IEEE Engineering in
Medicine and Biology Society (EMBC), pages 3130–
3133.
Shaffer, F. and Ginsberg, J. (2017). An overview of heart
rate variability metrics and norms. Frontiers in public
health, 5:258.
Tarvainen, M. P., Niskanen, J.-P., Lipponen, J. A., Ranta-
Aho, P. O., and Karjalainen, P. A. (2014). Kubios
hrv–heart rate variability analysis software. Computer
methods and programs in biomedicine, 113(1):210–
220.
Thayer, J. F.,
˚
Ahs, F., Fredrikson, M., Sollers III, J. J., and
Wager, T. D. (2012). A meta-analysis of heart rate
variability and neuroimaging studies: implications for
heart rate variability as a marker of stress and health.
Neuroscience & Biobehavioral Reviews, 36(2):747–
756.
Trevizol, A. P., Taiar, I., Barros, M. D., Liquidatto, B.,
Cordeiro, Q., and Shiozawa, P. (2015). Transcuta-
neous vagus nerve stimulation (tvns) protocol for the
treatment of major depressive disorder: A case study
assessing the auricular branch of the vagus nerve.
Epilepsy & Behavior, 53:166–167.
Vonck, K., De Herdt, V., and Boon, P. (2009). Vagal nerve
stimulation — a 15-year survey of an established treat-
ment modality in epilepsy surgery. In Advances and
Technical Standards in Neurosurgery, pages 111–146.
Springer Vienna.
BIOSIGNALS 2021 - 14th International Conference on Bio-inspired Systems and Signal Processing
168