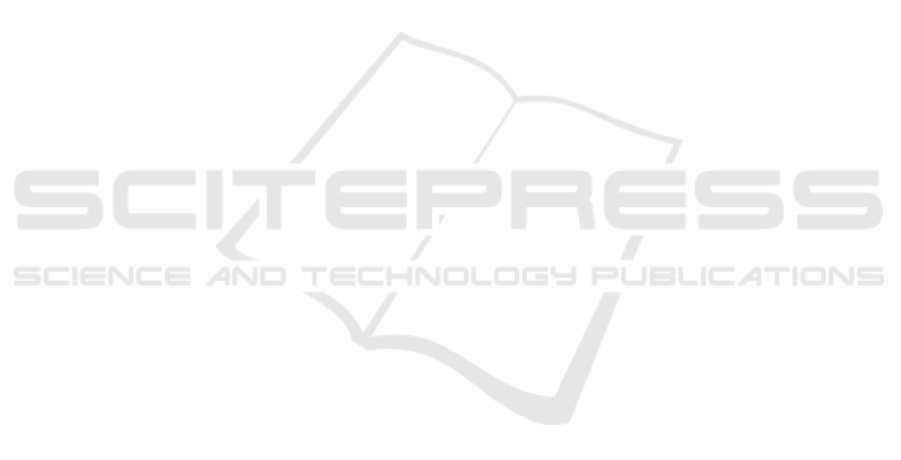
ACKNOWLEDGEMENTS
The research leading to these results has re-
ceived funding from RoboCity2030-DIH-CM Madrid
Robotics Digital Innovation Hub (”Rob
´
otica aplicada
a la mejora de la calidad de vida de los ciudadanos.
fase IV”; S2018/NMT-4331), funded by ”Programas
de Actividades I+D en la Comunidad de Madrid” and
cofunded by Structural Funds of the EU.
REFERENCES
Colorado, J., Barrientos, A., Rossi, C., and Breuer, K.
(2012). Biomechanics of smart wings in a bat robot:
morphing-wings using SMA actuators. Bioinspiration
& Biomimetics.
Colorado, J., Barrientos, A., Rossi, C., and Parra, C. (2013).
Inertial attitude control of a bat-like morphing-
wing air vehicle. Bioinspiration & Biomimetics,
8(1):016001.
Coral, W., Rossi, C., Colorado, J., Lemus, D., and Barri-
entos, A. (2012). Sma-based muscle-like actuation in
biologically inspired robots: A state of the art review.
In Berselli, G., Vertechy, R., and Vassura, G., editors,
Smart Actuation and Sensing Systems, chapter 3. Inte-
chOpen, Rijeka.
Coral, W., Rossi, C., Curet, O. M., and Castro, D. (2018).
Design and assessment of a flexible fish robot actuated
by shape memory alloys. Bioinspiration & biomimet-
ics, 13(5):056009.
Featherstone, R. (2008). An Architecture for Fast and
Accurate Control of Shape Memory Alloy Actua-
tors. The International Journal of Robotics Research,
27(5):595–611.
Hashimoto, M., Tabata, T., and Yuki, T. (1999). Develop-
ment of electrically heated SMA active forceps for la-
paroscopic surgery. IEEE International Conference
on Robotics and Automation Cat No99CH36288C,
3(May):2372–2377.
Hedenstr
¨
om, A., Johansson, L. C., and Spedding, G. R.
(2009). Bird or bat: comparing airframe design
and flight performance. Bioinspiration biomimetics,
4(1):015001.
Ho, M., McMillan, A. B., Simard, J. M., Gullapalli,
R., and Desai, J. P. (2011). Toward a Meso-
Scale SMA-Actuated MRI-Compatible Neurosurgical
Robot: Robotics, IEEE Transactions on. Robotics
IEEE Transactions on, PP(99):1–10.
Hunter, I. W. and Lafontaine, S. (1992). A comparison of
muscle with artificial actuators. In SolidState Sensor
and Actuator Workshop 5th Technical Digest IEEE,
pages 178–185.
Hunter, I. W., Lafontaine, S., Hollerbach, J. M., and Hunter,
P. J. (1991). Fast reversible NiTi fibers for use in mi-
crorobotics. In Micro Electro Mechanical Systems,
pages 166–170. Ieee.
Ikuta, K. (1990). Micro/miniature shape memory alloy ac-
tuator. In International Conference on Robotics and
Automation, volume 3, pages 2156–2161. IEEE Com-
put. Soc. Press.
Iriarte-D
´
ıaz, J., Riskin, D. K., Willis, D. J., Breuer, K. S.,
and Swartz, S. M. (2011). Whole-body kinematics
of a fruit bat reveal the influence of wing inertia on
body accelerations. Journal of Experimental Biology,
214(Pt 9):1546–1553.
Kuribayashi, K. (1986). A New Actuator of a Joint Mecha-
nism Using TiNi Alloy Wire. The International Jour-
nal of Robotics Research, 4(4):47–58.
Li, W. D., Guo, W., Li, M. T., and Zhu, Y. H. (2006). Design
and Test of a Capsule Type Endoscope Robot with
Novel Locomation Principle. 2006 9th International
Conference on Control Automation Robotics and Vi-
sion, 20(02):1–6.
Liu, X., Luo, H.-Y., Liu, S.-P., and Wang, D.-F. (2011). Pilot
study of SMA-based expansion device for transanal
endoscopic microsurgery. In 2011 International Con-
ference on Machine Learning and Cybernetics, pages
1420–1424. IEEE.
M. Hashimoto, Takeda, M., Sagawa, H., Chiba, I., and Sato,
K. (1985). Application of shape memory alloy to
robotic actuators. J. of Robotic Systems, 2(1):3–25.
Migamotors (2019). Migamotors Company, 2012, Avali-
able from: http://www.migamotors.com/.
Pons, J. L. (2005). Emerging Actuator Technologies: A Mi-
cromechatronic Approach. Wiley.
Raynaerts, D. and Brussel, H. V. (1991). Development of a
SMA high performance robotic actuator. In Fifth In-
ternational Conference on Advanced Robotics Robots
in Unstructured Environments, pages 61–66. Ieee.
Riskin, D. K., Iriarte-D
´
ıaz, J., Middleton, K. M., Breuer,
K. S., and Swartz, S. M. (2010). The effect of body
size on the wing movements of pteropodid bats, with
insights into thrust and lift production. Journal of Ex-
perimental Biology, 213(Pt 23):4110–4122.
Rossi, C., Colorado, J., Coral, W., and Barrientos, A.
(2011). Bending continuous structures with SMAs :
a novel robotic fish design. Bioinspiration biomimet-
ics, 045005(4):045005.
Rossi, C., Coral, W., and Barrientos., A. (2010). SMA Con-
trol for Bio-mimetic Fish Locomotion. In Interna-
tional Conference on Informatics in Control, Automa-
tion and Robotics (ICINCO), Madeira.
Shi, Z., Liu, D., Ma, C., and Zhao, D. (2011). Accurate con-
trolled Shape Memory Alloy Actuator for Minimally
Invasive Surgery. Self, pages 817–822.
Swartz, S. M., Bishop, K. L., and Ismael-Aguirre, M. F.
(2005). Dynamic complexity of wing form in bats:
implications for flight performance. Functional and
evolutionary ecology of bats, pages 110–130.
Waram, T. (1993). Actuator Design Using Shape Memory
Alloys. T.C. Waram.
Motor-less and Gear-less Robots: New Technologies for Service and Personal Robots
421