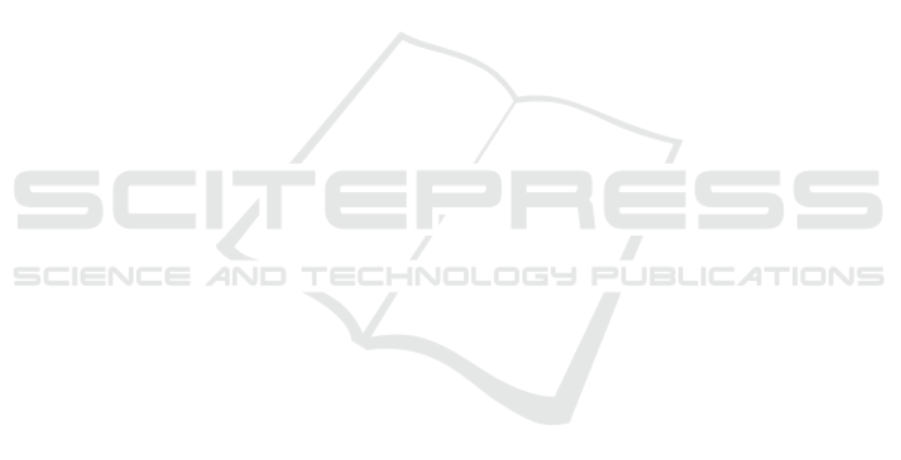
REFERENCES
Di Roberto, R., Nascetti, A., Paris, C., and Paolozzi, A.
(2015a). Optical payload for high-resolution Earth
imaging suitable for microsatellites. In 2015 IEEE
15th International Conference on Environment and
Electrical Engineering (EEEIC), pages 1863–1868.
IEEE.
Di Roberto, R., Paris, C., Nascetti, A., Paolozzi, A.,
and Graziani, F. (2015b). Assessment of a low-cost
multilayer insulation system for thermal control of
nanosatellites. In 66th International Astronautical
Congress. IAF.
Aliane, N., Martnez, A., Fraile, A., and Ortiz, J. (2007).
Labnet: A remote control engineering laboratory. In-
ternational Journal of Online Engineering (iJOE),
3(02).
Bosco, A., Cantone, C., Dell’Agnello, S., Monache, G. D.,
Franceschi, M. A., Garattini, M., Napolitano, T., Ciu-
folini, I., Agneni, A., Graziani, F., Ialongo, P., Lu-
cantoni, A., Paolozzi, A., Peroni, I., Sindoni, G., Bel-
lettini, G., Tauraso, R., Pavlis, E., Currie, D., Rubin-
cam, D., Arnold, D., Matzner, R., and Slabinski, V.
(2007). Probing gravity in NEO with high-accuracy
laser-ranged test masses. International Journal of
Modern Physics D, 16(12a):2271–2285.
Casini, M., Prattichizzo, D., and Vicino, A. (2001). The au-
tomatic control telelab: a remote control engineering
laboratory. In Decision and Control, 2001. Proceed-
ings of the 40th IEEE Conference on, volume 4, pages
3242–3247. IEEE.
Ciufolini, I., Monge, B. M., Paolozzi, A., Koenig, R., Sin-
doni, G., Michalak, G., and Pavlis, E. (2013). Monte
carlo simulations of the LARES space experiment to
test general relativity and fundamental physics. Clas-
sical and Quantum Gravity, 30.
Ciufolini, I., Paolozzi, A., and Paris, C. (2012a). Overview
of the LARES mission: orbit, error analysis and tech-
nological aspects. Journal of Physics. Conference Se-
ries, 354. conference 1.
Ciufolini, I., Paolozzi, A., Paris, C., and Sindoni, G.
(2014). The LARES satellite and its minimiza-
tion of the thermal forces. In 2014 IEEE Metrol-
ogy for Aerospace (MetroAeroSpace), pages 2014–5–
29/2014–5–30. IEEE.
Ciufolini, I. and Pavlis, E. C. (2004). A confirmation of the
general relativistic prediction of the Lense-Thirring
effect. Nature, 431:958–960.
Ciufolini, I., Pavlis, E. C., Paolozzi, A., Ries, J., Koenig, R.,
Matzner, R., Sindoni, G., and Neumayer, H. (2012b).
Phenomenology of the Lense-Thirring effect in the so-
lar system: measurement of frame-dragging with laser
ranged satellites. New Astronomy, 17:341–346.
ECSS (2002). ECSS-e-10-03a, space engineering: Test-
ing. , European Cooperation for Space Standardiza-
tion - ECSS Secretariat ESA-ESTEC Requirements
and Standards Division Noordwijk, The Netherlands.
ECSS (2012). ECSS-e-10-03a, space engineering: Test-
ing. , European Cooperation for Space Standardiza-
tion - ECSS Secretariat ESA-ESTEC Requirements
and Standards Division Noordwijk, The Netherlands.
Eslami, A., Williams, A., Lapat, L., Krauss, K., and Osareh,
A. R. (2008). A remote control project to enhance un-
dergraduate students interest and knowledge in indus-
trial automation. In Proceedings of IAJC-IJME Inter-
national Conference.
Gustavsson, I. (2003). A remote access laboratory for elec-
trical circuit experiments. International Journal of
Engineering Education, 19(3):409–419.
Jaggers, C. H., Meshishnek, M. J., and Coggi, J. M. (1993).
Thermal control paints on ldef: Results of m0003 sub-
experiment 18. In LDEF: 69 Months in Space. Part
3: Second Post-Retrieval Symposium; p 1075-1092.
NASA. Langley Research Center.
Macdonald, M. and Badescu, V. (2014). The International
Handbook of Space Technology. Springer.
Marco, J., Bhojaraj, H., and Hulyal, R. (2003). Evaluation
of thermal control materials degradation in simulated
space environment. In Fletcher, K., editor, Materials
in a Space Environment, volume 540 of ESA Special
Publication, pages 359–366.
May, D., Terkowsky, C., Haertel, T., and Pleul, C. (2013).
Bringing remote labs and mobile learning together.
International Journal of Interactive Mobile Technolo-
gies – iJIM, 7(3):54–62.
Paolozzi, A. and Ciufolini, I. (2013). LARES succesfully
launched in orbit: satellite and mission description.
Acta Astronautica, 91:313–321.
Paolozzi, A., Ciufolini, I., Felli, F., Brotzu, A., and Pilone,
D. (2009). Issues on LARES satellite material. In 60th
International Astronautical Congress - IAC, Daejeon,
Republic of Korea, number IAC-09.C2.4.5. IAF.
Paolozzi, A., Ciufolini, I., Paris, C., and Sindoni, G.
(2015a). LARES, a new satellite specifically designed
for testing general relativity. International Journal of
Aerospace Engineering, Volume 2015:9 pages. Arti-
cle ID 341384.
Paolozzi, A., Ciufolini, I., Paris, C., and Sindoni, G.
(2015b). Lares-lab: A thermo-vacuum facility for re-
search and e-learning - tests of lares satellite compo-
nents and small payloads for e-learning. In Proceed-
ings of the 7th International Conference on Computer
Supported Education, pages 467–474.
Paolozzi, A., Ciufolini, I., Paris, C., Sindoni, G., and Spano,
D. (2012a). Qualification tests on the optical retro-
reflectors of LARES satellite. In 63rd International
Astronautical Congress IAC 2012, Naples, Italy. IAF.
Paolozzi, A., Ciufolini, I., Paris, C., Spano, D., Battaglia,
G., and Reinhart, N. (2012b). Thermal tests on
LARES satellite components. In 63rd International
Astronautical Congress IAC 2012, Naples, Italy. IAF.
Paolozzi, A., Ciufolini, I., Schirone, L., Peroni, I., Paris,
C., Spano, D., Sindoni, G., Vendittozzi, C., Battaglia,
G., and Ramiconi, M. (2010). Tests of LARES cube
corner reflectors in simulated space environment (pre-
liminary results). In 61th International Astronautical
Congress - IAC.
Paolozzi, A., Ciufolini, I., and Vendittozzi, C. (2011). En-
gineering and scientific aspects of LARES satellite.
Acta Astronautica, 69:127–134.
Upgrade of the LARES-lab Remote Controllable Thermo-vacuum Facility - Lab Improvements for Remote Testing and e-Learning
351