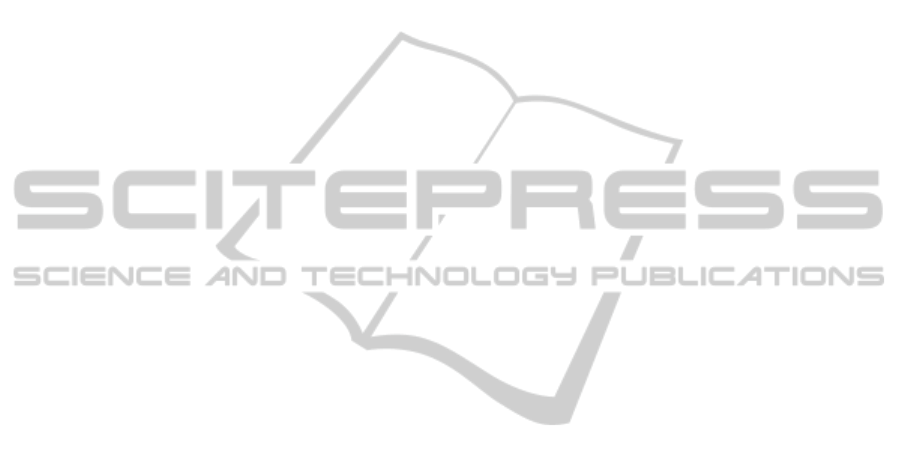
M. T. Islam , M. R. I. Faruque, N. Misran, “ Reduction of
Specific Absorption Rate (SAR) in the Human Head
With Ferrite Material and Metamaterial” Progress In
Electromagnetics Research C, Vol. 9, 4758, 2009.
H. Raad, A. Abbosh, H. Al-Rizzo, D. Rucker, "Flexible
and Compact AMC Based Antenna for Telemedicine
Applications," Antennas and Propagation, IEEE
Transactions on , vol.PP, no.99, pp.1, 0, 2013.
H. R. Khaleel, H. Al-Rizzo, D. Rucker, Y. Al-Naiemy,
"Flexible printed monopole antennas for WLAN
applications," Antennas and Propagation (APSURSI),
2011 IEEE International Symposium on , vol., no.,
pp.1334-1337, 3-8 July 2011.
H.-T. Chou, H.-C. Cheng, H.-T. Hsu, and L.-R. Kuo, “
Investigation of Isolation Improvement Techniques for
MIMO WLAN Portable Terminal applications”,
Progress In Electromagnetics Research, PIER 85,
349–366, 2008.
A. A. Abouda and S. G. Haggman, “Effect of Mutual
Coupling on Capacity of MIMO Wireless Channels in
High SNR Scenario”, Progress In Electromagnetics
Research, PIER 65, 27–40, 2006.
S. Alamouti, “A simple transmit diversity technique for
wireless communications,” IEEE J. Select. Areas
Commun., vol. 16, no. 8, pp. 1451–1458, Oct. 1998.
D. shan Shiu, G. J. Foschini, M. J. Gans, and J. M. Kahn,
“Fading correlation and its effect on the capacity of
multielement antenna systems,” IEEE Trans.
Commun, vol. 48, pp. 502–513, 2000.
H. Shin and J. H. Lee, “Capacity of multiple-antenna
fading channels: spatial fading correlation, double
scattering, and keyhole,” IEEE Transactions on
Information Theory, vol. 49, no. 10, pp. 2636–2647,
2003.
J. W. Wallace and M. A. Jensen, “Mutual coupling in
mimo wireless systems: a rigorous network theory
analysis,” IEEE Transactions on Wireless
Communications, vol. 3, no. 4, pp. 1317–1325, 2004.
P. Kyritsi, D. Cox, R. Valenzuela, and P. Wolniansky,
“Correlation analysis based on mimo channel
measurements in an indoor environment,” IEEE
Journal on Selected Areas in Communications, vol.
21, no. 5, pp. 713–720, 2003.
S. Caban and M. Rupp, “Impact of transmit antenna
spacing on 2x1 alamouti radio transmission,”
Electronics Letters, vol. 43, no. 4, pp. 198–199, 2007.
Ikeuchi, R.; Hirata, A., "Dipole Antenna Above EBG
Substrate for Local SAR Reduction," Antennas and
Wireless Propagation Letters, IEEE, vol.10, no.,
pp.904, 906, 2011.
C. Caloz, H. Okabe, T. Iwai, and T. Itoh, “A simple and
accurate model for microstrip structures with slotted
ground plane,” IEEE Microwave Wireless Comp. Lett.,
vol. 14, no. 4, pp. 133–135, Apr. 2004.
M. M. Bait-Suwailam, M. S. Boybay, and O. M. Ramahi,
“Electromagnetic coupling reduction in high-profile
monopole antennas using single-negative magnetic
metamaterials for mimo applications,” IEEE Trans.
Antennas Propag., vol. 58, no. 9, pp. 2894–2902, Sept.
2010.
J. Pendry, A. Holden, D. Robbins, and W. Stewart,
“Magnetism from conductors and enhanced nonlinear
phenomena,” IEEE Trans. Microw. Theory Tech., vol.
47, no. 11, pp. 2075–2084, Nov. 1999.
(2010, Apr.) CST microwave studio. [Online].
Available:http://www.cst.com/Content/Products/MWS
/Overview.aspx.
S. Blanch, J. Romeu, and I. Corbella, “Exact
representation of antenna system diversity
performance from input parameter description,”
Electronics Letters, p. 705707, May 2003.
H. R. Khaleel, H. Al-Rizzo, D. Rucker, "Compact
Polyimide-Based Antennas for Flexible
Displays," IEEE Display Technology, Journal of,
vol.8, no.2, pp.91-97, Feb. 2012.
H. R. Khaleel, H. Al-Rizzo, D. Rucker, S. Mohan, "A
Compact Polyimide-Based UWB Antenna for Flexible
Electronics," Antennas and Wireless Propagation
Letters, IEEE , vol.11, no., pp.564-567, 2012.
BIODEVICES2014-InternationalConferenceonBiomedicalElectronicsandDevices
44