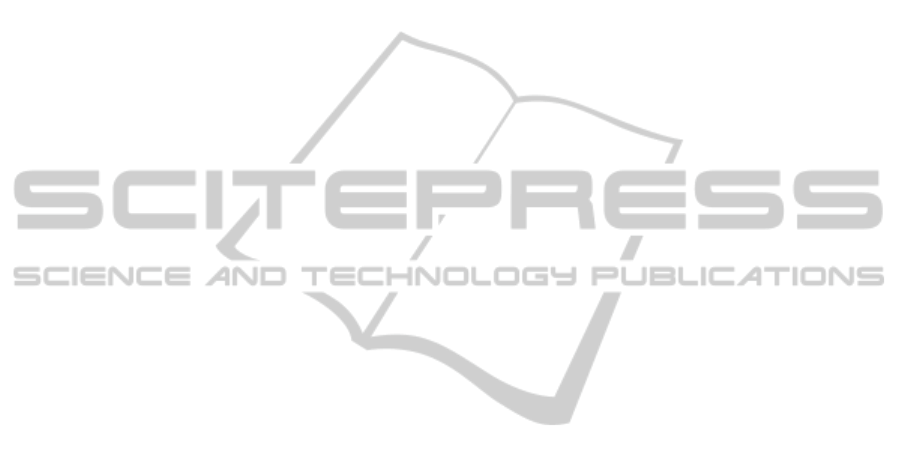
of plasticizer are demonstrated.
2 MATERIALS AND METHODS
Polymers for PNCC production were selectively
chosen. PI has been selected as highly non-polar
matrix with a purpose to detect non-polar VOC. On
the contrary PEG, PVAc was selected as highly
polar matrix to detect polar VOC like methanol,
ethanol, propanol, butanol, formamide, acetone,
methyl ethyl ketone etc. PVA was chosen for highly
polar solvent vapour presence determination. As
EVA copolymer contains non-polar part – ethylene
and polar part - 29.7% vinyl acetate, it was picked
up to produce composite, which could sense both
polar and non-polar solvents.
For all produced composites high structured
carbon black PRINTEX XE2 (mean diameter 30 nm,
DBP absorption - 380 ml/100 g, BET surface area -
950m
2
/g) as conductive filler was used. Firstly,
HSCB was dispersed in appropriate solvent using
Hielscher UP200S ultrasound homogenizer. Specific
power 1W/ml was applied for 5 minutes. Secondly,
the HSCB dispersion in solvent was added to a
polymer solution in the same solvent. Magnetic
stirring for 1 to 24 hours were applied. After
magnetic stirring polymer-HSCB mixture was
coated on epoxy laminate substrate with copper or
brass wire (in case of PI-NCC) electrodes. The
composite layer on epoxy laminate substrate was
obtained by repeated immersion of the epoxy
laminate into the mixture and subsequent solvent
evaporation from the film. Immersion-evaporation
cycles were repeated up to 4 times. PI-NCC after
solvent evaporation was cured under 30 atm pressure
at 150
o
C for 15 minutes. Brass wire electrodes were
selected for PI-NCC production because at the time
of curing chemical bonds between polyisoprene
rubber and brass wires are formed, which ensures
excellent composite adhesion to electrodes.
Produced samples were in size 10x14mm (width x
length) and with varying thickness.
For PVA-NCC production slightly different
production method was used. Firstly, PVA was
dissolved in water for 6h at 80
o
C. Secondly, HSCB
was dispersed in distilled water using Hielscher
UP200S ultrasound homogenizer. Specific power
2.5W/ml was applied for 5minutes. Thirdly, HSCB
suspension in water was added to 20ml of 10% PVA
solution in water. Obtained mixture was stirred with
glass beads for 10 minutes. The mixture was purred
out in Petri dishes. To obtain hydrogel from the
mixture, it was subjected to repeated freezing (12h at
-12
o
C) and thawing (12h at +25
o
C) cycles. Cycles
were repeated 3 times. Samples with dimensions
5x50x0.3mm were used for measurements. At the
time of freezing and thawing hydrogen crosslinks
are formed in PVA (Stasko, J., Kalnins, M., Dzene,
A., Tupureina, V., 2009). These physical crosslinks
prevents PVA from dissolution in water.
HSCB content in PI-NCC was varied form 2.2 to
6.6 phr (parts per hundred rubber). In case of PEG-
NCC, PVAc-NCC, EVA-NCC HSCB was held
constant 10 parts per hundred polymer (php) and
9php for PVA-NCC, respectively.
3 RESULTS AND DISSCUSION
3.1 Evaluation of Polymer Matrix
Structural State
PEG with molecular weight 40000 was used for
PEG-NCC production. Hydroxyl end groups in
chemical structure of PEG determine it polar nature
(ε =3.37), and single polymer backbone without side
branches ensures highly crystalline (92%) structure
formation.
PVAc with molecular weight 101600 was used
for PVAc-NCC production. PVAc has relatively
large acetate side groups, which act as steric
hindrance for three dimensional structure formation
of PVAc. As a result PVAc has amorphous
structure. EVA copolymer structure is composed of
ethylene and vinylacetate repeating units.
Introduction of vinylacetate in ethylene structure
leads to reduced copolymer crystallinity.
Differential scanning calorimetry (DSC) was used
for matrix material structural state analyzes, when it
is pure as well as in composite content. DSC
measurements were carried out only for composites
indented for detection of polar VOC (see Table 1).
Glass transition temperature (T
g
) and crystallinity
has been chosen as parameters for evaluation
because both greatly influence the composite VOC
sensitivity. T
g
indicate how flexible are polymer
macromolecules at room temperature. As lower
value of Tg as more rubbery like amorpous polymer
is and more flexible are polymer macromolecules.
Therefore more rapidly segmental motions of the
polymer can be performed by absorption of analyte.
VOC molecule absorption and polymer swelling
would be more favourable by hyperelastic than by
stiff and brittle matrix. Segmental motions can be
made more easily in amorphous structure than in
closely packed crystalline. It is seen in Table 1, that
crystallinity of EVA and PEG decreases, when
ATMOSPHERE CONTROL BY CHEMORESISTIVE POLYMER COMPOSITES
371