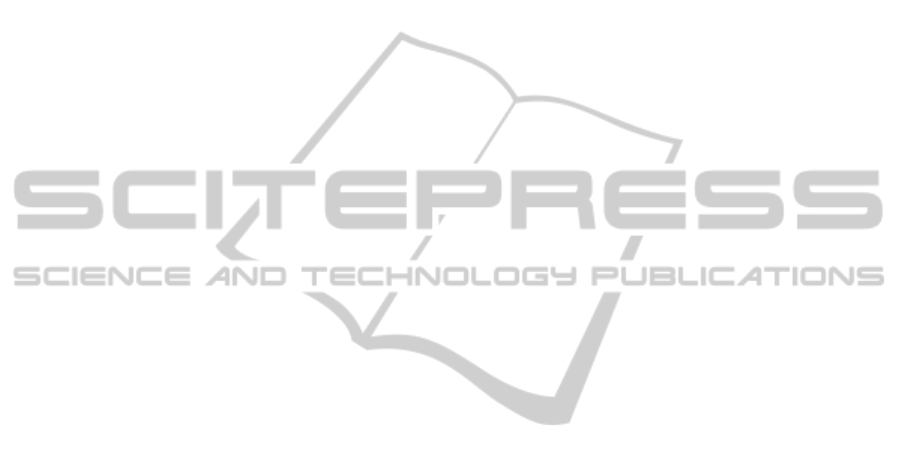
to be applied to real patients and help them to
improve their daily life.
However certain improvements can be made to
make the device more suitable for clinical purposes.
The pulse form could be changed to an alternating
form, since a non alternating pulse can cause toxic
reaction under the electrodes because of
accumulating ions under one of the electrodes.
However for testing purposes direct current can be
used since the time of current flowing is not very
long.
The acceptance of the skin to electrical
stimulation is also nonlinear. If a microprocessor is
integrated into the design an intelligent processing of
the sensor data is possible. This would improve the
feedback. The research group presenting this paper
is currently working in processing the data from the
Sensor Unit in an intelligent way using knowledge
based systems.
The next step in our research will be the
development of a more flexible system that is taking
into account the points mentioned above. It is hoped
that patients suffering from sensory loss can have an
enormous improvement in their daily life when
using an electrocutaneous feedback system, so they
can better walk, balance and perform tasks that
require the estimation of pressure applied to the foot.
ACKNOWLEDGEMENTS
The authors want to thank Angel Torres Perez and
Adam Wright for their help in the design and the
manufacturing of the circuit board, as well as Poole
Hospital to provide the facilities for the testing.
REFERENCES
Matjevic Z., Jensen P. L., 2008. Development and
evaluation of a two-dimensional electrocutaneous
cognitive feedback system for use in paraplegic
standing, Journal of Medical Engineering &
Technology, vol. 24, no. 5. pp. 215-226
Jeonghun K., Mraz R., Baker N., A Data Glove with
Tactile Feedback for fMRI of Virtual Reality
Experiments, CyberPsychology & Behavior, vol. 6,
no. 5, pp. 497, 2003.
Asamura, N.; Yokoyama, N.; Shinoda, H., 2002,
Selectively stimulating skin receptors for tactile
display, Computer Graphics and Applications, IEEE,
vol. 18, no. 6, pp. 32-37
Kuiken T. A., P. D. Marasco, B. A. Lock et al,. 2007.
Redirection of cutaneous sensation from the hand to
the chest skin of human amputees with targeted
reinnervation," 50, PNAS, pp. 20061-20066.
Maheshwari V., and R. F. Saraf, 2008. Tactile Sensing To
Sense on a Par with Human Finger. Angew. Chem.
Int. Ed., pp. 7808-7826.
Cotton J., I. M. Graz, and S. P. Lacour. 2009. A
Multifunctional Capacitive Sensor for Stretchable
Electronic Skins. Ieee Sensors Journal, vol. 9, no. 12,
pp. 2008-2009..
Arshak K., E. Jafer, and A. Fox, 2005. Design of a new
thick film capacitive pressure and circuitry interface.
Composites Science and Technology, vol. 65, no. 5,
pp. 757-764.
Hollinger A., Wanderley M., 2006, Evaluation of
Commercial Force-Sensing Resistors, Nime06
Herrera-May, A. L. 2009, Electromechanical analysis of a
piezoresistive pressure microsensor for low-pressure
biomedical applications, Revista Mexicana De Fisica,
vol. 55, no. 1, p. 14-24
Zhang D., Guan T.H., 2007. Functional Electrical
Stimulation in Rehabilitation Engineering: A Survey.
Proceedings of the International Convention on
Rehabilitation Engineering & Assistive Technology.
pp. 221-226.
Sheffler L. R., and J. Chae. 2007. Neuromuscular
electrical stimulation in neurorehabilitation. Muscle &
Nerve, vol. 35, no. 5, pp. 562-590. Robertson, PhD,
Alex Ward, PhD, John Low, BA(Hons),
Electrotherapy Explained, 4th Edition - Principles and
Practice, BUTTERWORTH HEINEMANN , MAY-
2006
Lundborg G., B. Rosen, K. Lindstrom, 1998. Artificial
sensibility based on the use of piezoresistive sensors -
Preliminary observations. Journal of Hand Surgery-
British and European Volume, vol. 23B, no. 5, pp.
620-626
BIODEVICES 2011 - International Conference on Biomedical Electronics and Devices
390