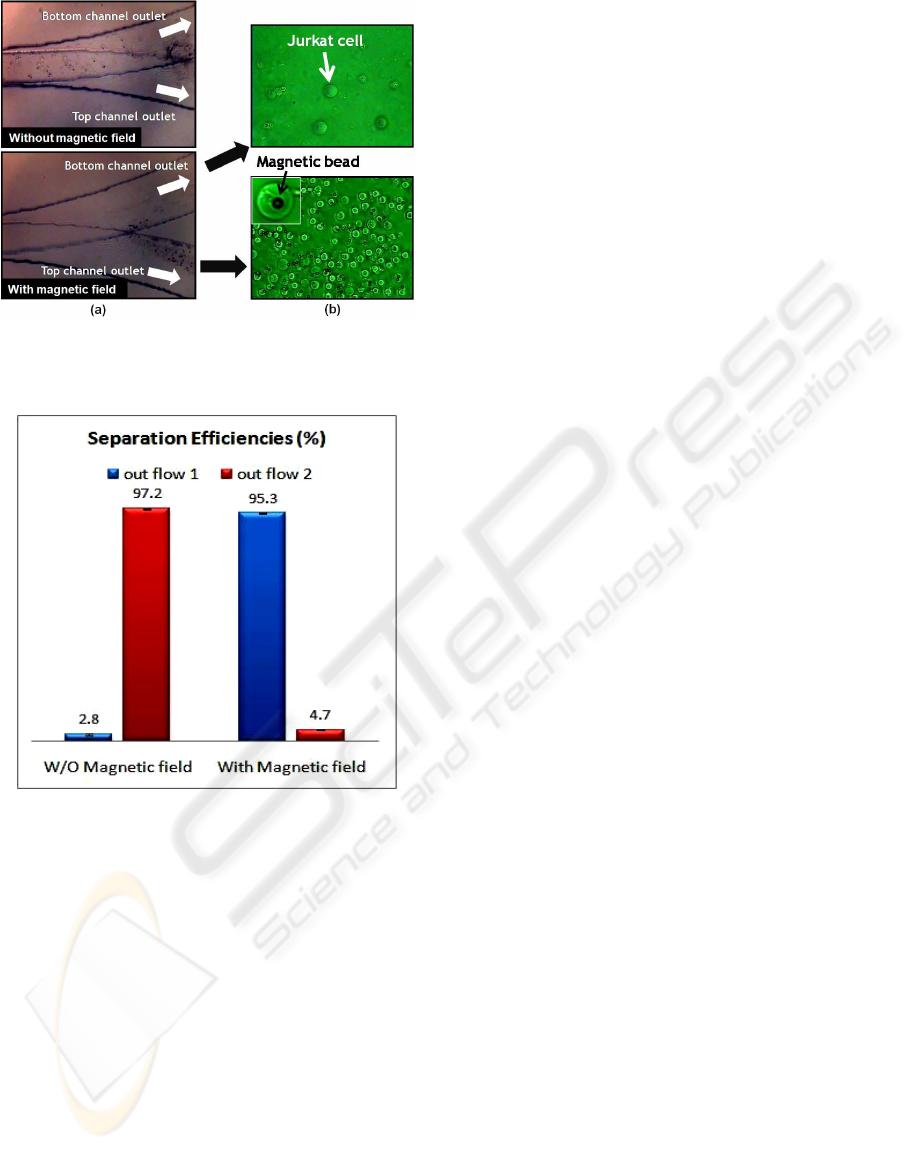
Figure 5: (a) Photographic image of microfluidic channel
(b) Photographic image of Jurkat cells and magnetic beads
from outlet of the channel.
Figure 6: Experimental results of the separation
efficiencies.
4 CONCLUSIONS
A new multi-layered channel for separating cells has
been introduced using microelectromagnet. Our
experiments demonstrate that specific cells can be
separated simply using a multi-layered microfluidic
channel with high efficiencies.
The efficiency of the separation by our approach
was comparable with that of conventional
magnetophoretic cell sorters (Bu at al. 2008)
(Smistrup at al. 2005). Our results identify a new
multi-layered microfluidic channel to isolate cells
for drug discovery and Lab-on-a-chip system
because of its attractive features such as high
throughput, continuous sorting, simply and rapidly
fabricated system.
ACKNOWLEDGEMENTS
This work was supported by National Core Research
Center (NCRC) for Nanomedical Technology of the
Korea Science & Engineering Foundation (Grant no.
R15-2004-024-01001-0), Seoul Research &
Business Development (R&BD Program, 11128)
and Korea Research Foundation Grant funded by the
Korean Government (MOEHRD) (KRF-2007-313-
D00073).
REFERENCES
Inglis, D., Riehn, R., Austin, R., & Sturm, J. 2004.
Continuous microfluidic immunomagnetic cell
separation. Applied Physics Letters, 85, 5093.
Pamme, N., & Wilhelm, C. 2006. Continuous sorting of
magnetic cells via on-chip free-flow magnetophoresis.
[Article]. Lab on a Chip, 6(8), 974-980.
Wolbers F, Andersson H, van den Berg A, Vermes I. 2004.
Apoptosis induced kinetic changes in autofluorescence
of HL60 cells-possible application for single cell
analysis on chip. Apoptosis 9:749–755
Pamme N. 2006. Magnetism and microfluidics. Lab Chip
6:24–38
Sun L, Zborowski M, Moore LR, Chalmers JJ. 1998.
Continuous, flow-through immunomagnetic cell
sorting in a quadrupole field. Cytometry 33:469–475
Kim, H., Son, O., Kim, K., Kim, S., Maeng, S., & Jung, H.
2007. Separation of apoptotic cells using a
microfluidic device. Biotechnology Letters, 29(11),
1659-1663.
Qasem R, Victor S, Daniel P, Chen Y. 2004. On-chip
microelectromagnets for magnetic-based bio-
molecules separation. J Magnetism and Magnetic Mat
281:150–172
Williams PS, Zborowski M, Chalmers JJ. 1999. Flowrate
optimization for the quadrupole magnetic cell sorter. Anal
Chem 71:3799–3807
Lagae L, Wirix-Speetjens R, Liu CX, Laureyn W, Borghs
G, Harvey S, Galvin P, Ferreira HA, Graham DL,
Freitas PP, Clarke LA, Amaral MD. 2005. Magnetic
biosensors for genetic screening of cystic fibrosis.
IEEE Proc Circuit Device Syst 152:393–400
Bu, M., Christensen, T. B., Smistrup, K., Wolff, A., &
Hansen, M. F. 2008. Characterization of a
microfluidic magnetic bead separator for high-
throughput applications. Sensors and Actuators, A:
Physical, 145-146(1-2), 430-436.
Smistrup, K., Hansen, O., Bruus, H., & Hansen, M. 2005.
Magnetic separation in microfluidic systems using
microfabricated electromagnets-experiments and
simulations. Journal of Magnetism and Magnetic
Materials, 293(1), 597-604.
A MULTI-LAYERED MICROFLUIDIC DEVICE FOR MAGNETOPHORETIC CELL SEPARATION
289