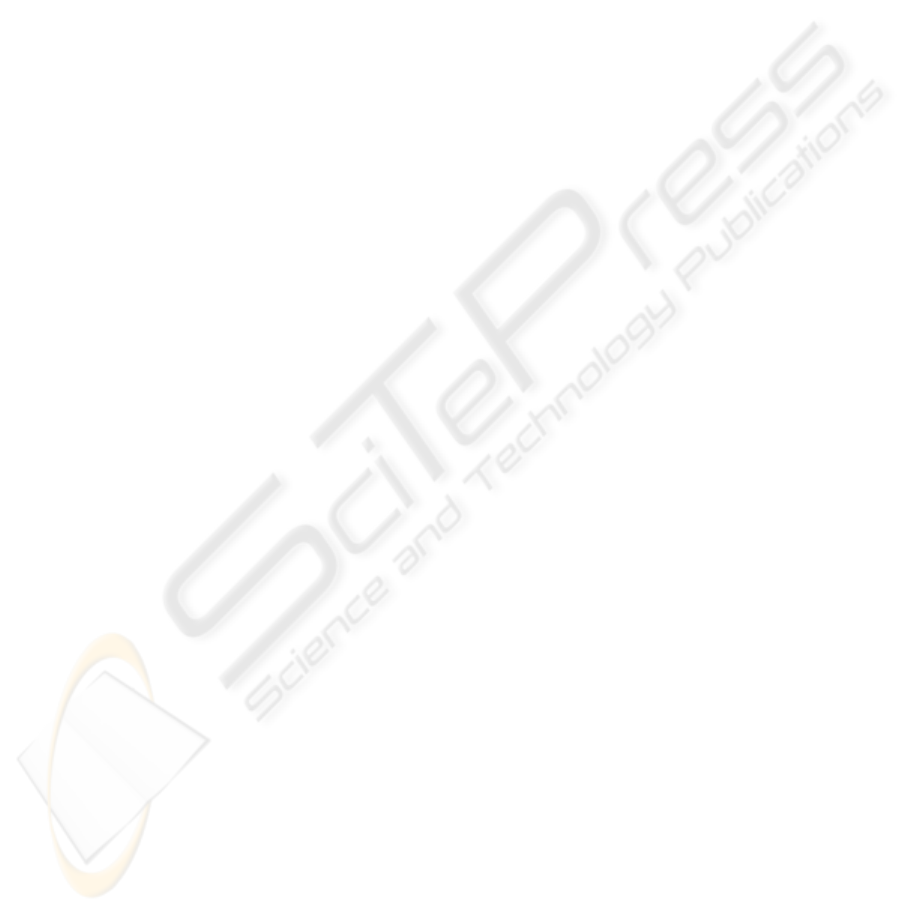
50% of current drugs are directed to GPCRs (Nambi
and Aiyar, 2003).
The number of known GPCRs is in the
thousands, and many more are being discovered as a
result of recent advances in genomics and
proteomics. To be useful for drug design, structures
of these drug targets should be elucidated, in order
to employ them by methods of “Structure Based
Drug Design” (SBDD). The structural aspects of
GPCRs are however a source of constant debate in
recent years (Bissantz et al., 2003).
Direct experimental study of GPCR structures is
currently too complicated due to their native
membrane environment. Until November 2007,
only a single G-protein-coupled receptor, bovine
rhodopsin, has been studied by high-resolution
crystallography (Palczewski et al., 2000; Okada and
Nakamichi, 2004). β2-adrenergic receptor was the
second GPCR to solve and its structure revealed fair
similarity to the model obtained based on rhodopsin
as a template (Rasmussen et al., 2007; Cherezov et
al., 2007). The prospects for elucidating the
structures of other GPCR are not very high, and
await a major breakthrough. With no other structures
at hand, rhodopsin and/or β2-adrenergic receptor are
considered to be the prototypes of the main family of
GPCRs, of type A.
Due to the lack of experimental 3D-structures of
other GPCRs, one could hope to gain from
approximations based on molecular models. While
“ab initio” modeling is not practical yet for any
protein, “homology” or “comparative” modeling are
quite established methods (Rayan et al., 2000) and
are expected to be especially successful in the GPCR
subfamily A, that is considered to have the general
features of rhodopsin. Indeed, many GPCR
structures have been modelled recently, based on the
template of bovine rhodopsin/β2-adrenergic
receptors, by using its backbone coordinates and
adding the appropriate side chains of each sequence
(Eszter and Zsolt, 2008). Such “homology” or
“comparative” modeling of GPCRs has been aided
mainly by experimental information from point
mutations and other experimental resources
(http://www.gpcr.irg/7tm/, 2006). The length of
helices in the TMD remain similar in the modelled
GPCRs to those of the template rhodopsin, and loops
are not included in the template construction, except
in those rare cases where loop lengths are similar to
those of rhodopsin. But other approaches for
constructing models of GPCRs suggest that GPCRs
could differ in their structure from rhodopsin even
though their general features are similar (Oliveira et
al., 2002).
There are a few indications to justify such
deviations from the rhodopsin structure, in
constructing models for other GPCRs. A review by
Baker and Sali (Schacham et al., 2001) has shown
that a homology model for a protein at medium size
at least and with sequence identity of less than 30%
to the template crystal structure is unreliable. The
averaged sequence identity of bovine rhodopsin to
hGPCRs is less than 20%, meaning a homology-
based approach is unlikely to provide a reliable
structure to be used for making predictions. Others
in the community think that this “rule” is correct in
globular proteins and it is doubtful if this “rule”
could be extended to membrane proteins. Also, this
rule does not specify how identity should be
distributed along a sequence. As much as the GPCRs
super family is united by an overall structural
topology and an ability to recruit and regulate the
activity of G proteins, sequence identity between
super family members, even in the more conserved
transmembrane cores is too low. Significant
sequence conservation is found, however, within
several subfamilies of GPCRs. The subfamily of
rhodopsin-like GPCRs is by far the largest (more
than 85% of GPCRs) and is characterized by the
presence of some 35 (out of ~190) highly conserved
residue positions in the TMD, that may be involved
in binding and/or in activation (Baler and Sali,
2001).
The conserved positions along the TM sequences
constitute less than 20%. In contrast, the intracellular
and extracellular loops and the N- and C- terminals
of GPCRs vary in their lengths and therefore they
pose an alignment problem. Palczewski, K. and his
colleagues (Baldwin et al., 1997) via investigation of
sequence analysis of the TMD of GPCRs
demonstrated that “… the extracellular domain is the
least conserved, while GPCRs display considerable
conservation toward the endoplasmic side...” While
this is an important observation, it lacks specific
quantitative character. The conclusions of that study
concentrated on individual residue conservation and
on microenvironment conservation, and have thus
detected the most conserved residues in the TMD.
The authors concluded by suggesting that “It is
reasonable to speculate that the overall fold of these
receptors is highly conserved”. One of the
implications of that study are thus, that it is
reasonable to use the overall structure of rhodopsin
to model the TMD of other GPCRs.
Therefore, the question remains open, to what
extent is the structure of rhodopsin useful as a
template for constructing models of other GPCRs? A
quantitative measure of conservation in that family
BIOSIGNALS 2009 - International Conference on Bio-inspired Systems and Signal Processing
292